« Prev Next »
The family of ERBB receptors (ERBB1–4) is involved in many key cellular functions, including cell growth, division, migration, adhesion, and apoptosis, which makes them fundamental to the proper physiological function of vertebrate tissue. Consequently, abnormal signaling can lead to disease, such as cancer. Like many receptors, their function can be modulated, depending on cellular context, a phenomenon called regulation. From their synthesis process along their journey to the cell membrane, to their activation and destruction, regulation of ERBB function happens at every step. What follows is a discussion of ERBB receptor regulation and the processes critical for their proper production and signaling.
What Are the Main Categories of ERBB Regulation?
Regulation occurs "from the cradle to the grave," that is, from the time the receptor proteins are initially created inside the cell to the remainder of their life cycle in the membrane and through their degradation. Regulation of ERBB function can occur by specific placement in subregions of the cell membrane as well as during its movement between the cell surface and the cytoplasm. Once the receptor is synthesized and sent to the cell membrane, it will stay there for some amount of time, after which it may return to the cell for recycling (and ultimate membrane return into another subcellular compartment) or become slated for degradation. In addition to regulation of subcellular location, ERBB receptors are also regulated in ways that affect their signaling activity. Ultimately, the maturation, dimerization, activation, time of existence (referred to as half-life), and degradation are all tightly regulated by either ERBB receptors themselves or other proteins and molecules.
The Maturation of an ERBB Receptor
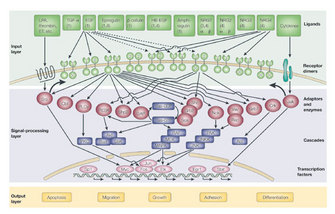

For a protein to become mature, it often needs help. Chaperones are proteins that, as their name implies, help prevent unwanted interactions among proteins. They bind to the protein they are helping and can assist with folding to prevent the protein from folding improperly; they can also assist in maturation by protecting the protein as it goes from the nascent to the mature state. Chaperones can also help the protein once it has been folded, and they stay with it for the majority of its life cycle.
Chaperones and ERBB Receptors
How was this mechanism of chaperones and ERBBs first understood? A specific class of drugs — benzoquinoid ansamycins, which include the drugs herbimycin A and geldanamycin — helped make it clear. These drugs are useful for research purposes because they inhibit several tyrosine kinases. They do so indirectly, by preventing the chaperone HSP90 from associating with the protein it is assisting (known as its client). In fact, these drugs are specifically HSP90 inhibitors. In 1994, P. Miller and colleagues looked at what would happen to cellular ERBB2 levels when cells were treated with herbimycin A (Miller et al. 1994). They used cells that normally express ERBB2, and they added the HSP90 inhibitor to these cells for increasing lengths of time. They then collected the contents of these calles (lysates) after each treatment period and measured the amount of ERBB2 remaining. Interestingly, they found that when cells were treated with herbimycin A, the level of ERBB2 protein diminished over time. This pivotal observation was the first indication that HSP90 could play a potent role in the life cycle of ERBB receptors, and more evidence about the interaction of HSP90 and other ERBBs was soon to come. Similarly, M. Sakagami and colleagues showed that HSP90 inhibitors caused depletion of EGFR, the most well known ERBB, over time (Sakagami, Morrison & Welch 1999). Through similar experiments, researchers have shown that all the ERBB receptors have some dependence on HSP90; that is, removal of HSP90 by an inhibitor causes depletion of the ERBB receptor in the cell.
Although HSP90 is a protective chaperone for all ERBB receptors, it can actually influence the function of one ERBB. This interaction was discovered by A. Citri and colleagues, who examined ERBB dimerization while inhibiting HSP90 (Citri et al. 2004). Researchers had established that, during activation of ERBB receptors, ERBBs can dimerize and become phosphorylated, which causes a series of downstream signals to other proteins inside the cell. In the HSP90 inhibition experiment, researchers focused on ERBB2 and found that HSP90 would detach from ERBB2, causing dimerization and an increase in downstream signaling. Furthermore, the addition of ligand for the ERBB2 dimerization partner, ERBB3, led to dissociation of HSP90 from ERBB2. These results suggested that HSP90 has a normal role in ERBB2 function, by inhibiting it from interacting spontaneously with other ERBB receptors. Thus, HSP90 does not completely interfere with ERBB2 functioning, but rather acts as a kind of gating mechanism for ERBB2 activation by preventing it from easily bumping into another receptor and activating. This finding is only one example of an intermolecular interaction that regulates ERBB receptors. However, many parts of the ERBB receptors themselves can influence regulation.
What Properties Intrinsic to the Receptors Regulate Their Activity?
As those who study ERBB activation have well characterized, dimerization of ERBB receptors leads to one receptor activating its partner and causes phosphorylation of tyrosines on the cytoplasmic end of the receptors. This phophorylation becomes a potent signal to a variety of intracellular proteins. As such, dimerization is a means of regulating activation. A good example exists in the interaction of ERBB2 and ERBB3. Alone, each receptor is not very potent; ERBB2 binds no known ligand, and ERBB3 has a minimally active kinase domain. But when these two receptors dimerize, their combination initiates a strong mitogenic signal for the cell (leading to mitosis) (Figure 2).
Indeed, there are distinct portions of these receptors involved in regulation of their activity, including the extracellular, transmembrane, cytoplasmic juxtamembrane (inside the cell and between the transmembrane and kinase domains), and kinase domains. Another area involved in regulation is the cytoplasmic tail, which harbors the tyrosines that signal through their phosphorylation. Discussion of these regulatory components is beyond the scope of the current discussion, but they bear mentioning as mechanisms that also fine-tune regulation.


What Are the Brakes on Activation?
Once activated, receptors cannot stay in an active state for too long or they would be constantly signaling, which would lead to aberrations in the cell and ultimately disease. To avoid that, the cell has developed different means of turning off or altering these signals. What methods do they use? Phosphorylation of receptors is the primary way they signal intracellularly, so if phosphorylation were reversed, then signaling would be inhibited. Reversing phosphorylation can be achieved through proteins called phosphatases, which remove phosphates.
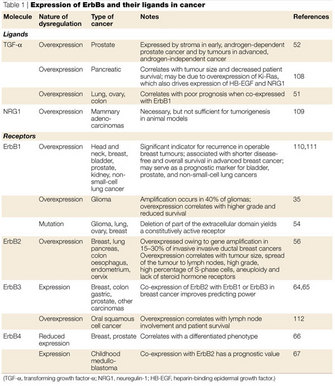

One important signaling mechanism related to internalization regulates the lifetime and signaling capabilities of these receptors. This mechanism, called ubiquitination, is the process by which a small protein call ubiquitin is attached to another protein and serves as a signal for the protein to be routed toward a certain path within the cell, such as to a lysosome or to the proteasome. One or several ubiquitin molecules can be added to proteins, and variations on this number and location of added ubiquitins will affect the interaction of the ubiquitinated protein with other cellular components, leading to different routing outcomes. Although ubiquitination is clearly an important means of directing receptors along certain paths in the cell, interactions with other proteins are also crucial for internalization. And, depending on what cellular context is for a receptor (e.g., what ligand is used, what molecules it interacts with, and what other receptors are coexpressed and at what levels), the ultimate fate of that receptor will differ. Down-regulation fates include dephosphorylation, recycling, routing to another area of the cell, degradation, and any combination thereof, and they vary greatly between different ERBB receptors. How each receptor's ever-changing cellular environment dictates its fate, and the mechanism by which each are internalized, is still unclear. These multifaceted complex mechanisms are fascinating, and many open questions regarding them remain. Due to the known aberrant expression patterns of ERBBs in certain cancers, this research has particular relevance to human disease (Table 1).
Summary
Due to their activation by growth factors, ERBBs are crucial to normal cellular function. Therefore, it is not surprising that their regulation mechanisms are complex. There are many means of regulating their signaling and their very presence in the membrane, and these regulation processes are largely dependent on the proteins they associate with and properties intrinsic to the receptors themselves. These regulatory mechanisms also help provide the great variety of signaling outcomes through ERBB receptors after ligand binding as well as prevent the dangerous state of indefinite signaling. Researchers are still learning about what other proteins are involved in regulating activation, maturation, and degradation of these receptors. The mechanisms by which these proteins and different sections of the receptors themselves regulate signaling are also areas ripe for more research.
References and Recommended Reading
Baulida, J. et al. All ErbB receptors other than the epidermal growth factor receptor are endocytosis impaired. Journal of Biological Chemistry 271, 5251–5257 (1996).
Carpenter, G. & Cohen, S. 125I-labeled human epidermal growth factor: Binding, internalization, and degradation in human fibroblasts. Journal of Cell Biology 71, 159–171 (1976).
Citri, A. et al. Hsp90 restrains ErbB-2/HER2 signalling by limiting heterodimer formation. EMBO reports 5, 1165–1170 (2004) doi:10.1038/sj.embor.7400300.
Citri, A. et al. Hsp90 recognizes a common surface on client kinases. Journal of Biological Chemistry 281, 14361–14369 (2006) doi:10.1074/jbc.M512613200.
Huang, F., Goh, L. K. & Sorkin, A. EGF receptor ubiquitination is not necessary for its internalization. Proceedings of the National Academy of Sciences 104, 16904–16909 (2007) doi:10.1073/pnas.0707416104.
Landau, M. & Ben-Tal, N. Dynamic equilibrium between multiple active and inactive conformations explains regulation and oncogenic mutations in ErbB receptors. Biochimica et Biophysica Acta 1785, 12–31 (2008) doi:10.1016/j.bbcan.2007.08.001.
Madshus, I. H. & Stang, E. Internalization and intracellular sorting of the EGF receptor: A model for understanding the mechanisms of receptor trafficking. Journal of Cell Science 122, 3433–3439 (2009) doi: 10.1242/jcs.050260.
Marmor, M. D. & Yarden, Y. Role of protein ubiquitylation in regulating endocytosis of receptor tyrosine kinases. Oncogene 23, 2057–2070 (2004) doi:10.1038/sj.onc.1207390.
Miller, P. et al. Depletion of the erbB-2 gene product p185 by benzoquinoid ansamycins. Cancer Research 54, 2724–2730 (1994).
Sakagami, M., Morrison, P. & Welch, W. J. Benzoquinoid ansamycins (herbimycin A and geldanamycin) interfere with the maturation of growth factor receptor tyrosine kinases. Cell Stress and Chaperones 4, 19–28 (1999).
Sorkin, A., Di Fiore, P. P. & Carpenter, G. The carboxyl terminus of epidermal growth factor receptor/erbB-2 chimerae is internalization impaired. Oncogene 8, 3021–3028 (1993).
Sorkin, A. & Goh, L. K. Endocytosis and intracellular trafficking of ErbBs. Experimental Cell Research 314, 3093–3106 (2008) doi:10.1016/j.yexcr.2008.07.029.
Wang, Y. N. et al. Nuclear trafficking of the epidermal growth factor receptor family membrane proteins. Oncogene 29, 3997–4006 (2010) doi:10.1038/onc.2010.157.
Warren, C. M. & Landgraf, R. Signaling through ERBB receptors: Multiple layers of diversity and control. Cellular Signalling 18, 923–933 (2006) doi:10.1016/j.cellsig.2005.12.007.
Yarden, Y. & Sliwkowski, M. X. Untangling the ErbB signalling network. Nature Reviews Molecular Cell Biology 2, 127–137 (2001) doi:10.1038/35052073.