« Prev Next »
In multicellular organisms, cells adopt social behaviors. Within a given organ or tissue, cells act in coordination in response to environmental cues. Have you ever seen the beautifully synchronized movements of threatened groups of shoaling fish? Cells behave in a similar fashion in processes as diverse as organogenesis and tissue regeneration. This coordinated behavior is partly dependent on signals delivered by adhesion receptors, which contribute to the onset of developmental genetic programs that occur synchronously in tissues and organs.
Adhesion Receptors: More Than Just Sticky Molecules
Our senses enable us to interact with the surrounding environment. Among them, touch can be defined as perception through physical contact. Upon stimulation, somatosensory receptors, which are involved in tactile sensitivity, trigger a series of events leading to an accurate perception of the immediate environment and causing an appropriate response to it. Think of a cell as an independent functional unit in the social context of a multicellular organism. Would not the cell's perception of its extracellular surroundings be relevant to establish appropriate connections with other cells, to provide orientation in three-dimensional space, and to participate in coordinated morphogenetic and regenerative processes? Adhesion receptors (ARs) fulfill most of these requirements in cells.
There is no doubt that ARs are "sticky" molecules, as we
briefly mention below, but ARs also contribute signals that allow cells to
migrate, proliferate, and survive. ARs act as part of the developmental
programs affecting all cells in a given organ or tissue in a timely and
coordinated fashion. ARs generate and transduce important signals to provide
accurate spatial information. These signals are essential for cell migration
during embryogenesis and growth arrest at defined stages during organogenesis
as well as for the vigorous cell proliferation that occurs in regenerating
tissues responding to injury.
Decades of experimental evidence show that ARs have dual roles. In one, ARs act as glue that holds cells together and attaches cells to the extracellular matrix. But also in their other role they function as molecular sensors, which provide spatial information that profoundly affects key cellular responses. These cellular responses range from motility to proliferation and survival. As we see below, many investigators have worked to experimentally dissect these two equally important functions of ARs since the mid-1980s.
Setting the Stage of Adhesive Interactions
In both experimental animals and in patients, the inactivation of ARs is incompatible with life unless the given AR has a restricted tissue distribution. For example, a malfunction of the α6β4 integrin that connects epithelial cell sheets to the basal membrane leads to a severe blistering disease called epidermolysis bullosa. In Glanzmann's thromboasthenia, defective expression of the platelet-specific integrin αIIbβ3 impairs platelet aggregation, resulting in a severe bleeding disorder. Finally, in leukocyte adhesion deficiency-1 (LAD-1), leukocytes fail to express their specific integrins (in the β2 family) and are unable to adhere to and cross blood vessels, which results in the inability of leukocytes to clear infections in many tissues (Wehrle-Haller & Imhof 2003). Remarkably, bacterial skin infections in LAD patients do not lead to pus formation because pus results from the accumulation of dead leukocytes at the site of an infection. Now, let us return to our starting assumption, that besides providing adhesion, ARs also convey signals that are important for many essential cellular responses. How did scientists discover these additional functions?
Adhesion Receptors: An Evolutionary Perspective
If ARs were major determinants of the social behavior of cells in multicellular organisms, it would be fair to hypothesize that they have coevolved with metazoans. Is that actually the case? Let us focus on the two main families of ARs, integrins and cadherins, which are engaged in cell-extracellular matrix and cell-cell adhesion, respectively. Indeed, integrins generally appear in early metazoans, such as the sponge Geodia cydonium, although sequences homologous to their conserved extracellular domains are found in prokaryotes (Johnson et al. 2009). These data suggest that the ligand-binding motifs of integrins were selected early in animal evolution. However, their adhesive function, which entails spanning the plasma membrane and indirectly linking to cytoskeletal elements, is a unique feature in metazoans.
The major cadherin families also appear in metazoans, and scientists have learned they are the main molecules responsible for segregating homologous cells away from one another (Gumbiner 2005). When scientists performed in vitro experiments that mixed cells expressing either cadherin X or Y in a single cell suspension, they observed that the cells readily formed homotypic clusters, meaning that the cadherin X-expressing cells segregated away from the cadherin Y-expressing cells (Takeichi 1988). Using inductive reasoning, scientists speculated that this phenomenon was the basis for tissue and organ morphogenesis, which is the main distinctive feature of multicellular organisms. By studying cadherins, scientists proposed this mechanism for tissue and organ morphogenesis long before the responsible coding genes were identified, comparatively analyzed, and manipulated using DNA recombination in model organisms (Takeichi 1988).
Stop and Go Signals Originating from Adhesion Receptors Control Proliferation
Scientists observed for years that when normal cells grow on a substratum made of extracellular matrix proteins, their proliferation is "anchorage-dependent." Anchorage to the substratum is an integrin-mediated adhesion process. If the cells are detached from the substratum, not only do they fail to respond to growth factors, but they actually undergo a form of apoptosis that has been named anoikis (from the Greek word "homelessness") (Frisch & Screaton 2001). Conversely, when cells growing in culture reach confluence and establish cadherin-dependent intercellular adhesions, they become "contact-inhibited"; that is, they immediately exit the cell cycle and enter a state of quiescence (Nelson & Daniel 2002). What regulates these stop and start signals?
Scientists postulated that ARs delivered signals that influenced the cells' proliferative responses to growth factors. Through careful observations and experiments, they learned that the "start" signals are delivered by integrins and the "stop" signals are mediated by cadherins. Strikingly, both of these responses are completely lost in tumor cells, which show anchorage-independent growth and proliferate freely and endlessly on top of one another (with no contact inhibition). Hence, understanding the nature of these AR signals can help us understand how normal cells are transformed into cancerous cells.
Signaling by Adhesion Receptors
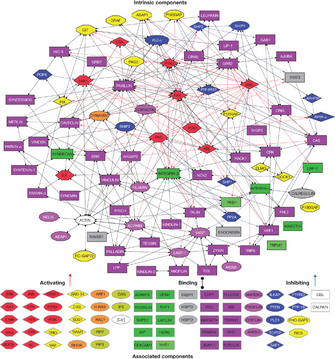

Scientists ruled out these possibilities by showing that ARs do not possess catalytic domains. In 2008, one research group identified a nonreceptor tyrosine kinase that associated constitutively with integrin-containing adhesion sites and was directly activated by integrin-mediated adhesion. To emphasize its unique subcellular localization, the kinase was named the focal adhesion kinase (FAK) (Parsons et al. 2008). Interestingly, upon activation, FAK is able to recruit a host of signaling adaptors, including Grb2 and Sos, which are shared by well-characterized signal transducing receptors. Thus, the activation of FAK leads to the activation of downstream pathways, which overlap with those triggered by growth factors, and ultimately leads to the onset of DNA replication (Juliano 2002; Parsons et al. 2008).
Scientists then learned that FAK does not interact directly with integrins, but rather that it does so indirectly through peripheral adaptor proteins, such as paxillin or α-actinin, which connect integrins to the actin cytoskeleton. Thus, integrins could behave as scaffolds by assembling large molecular networks that connect the plasma membrane to the actin cytoskeleton at sites of adhesion. These scaffolds could produce a local enrichment of signaling adaptors and effectors otherwise shared by other common signal transducing molecules, such as receptor tyrosine kinases (RTK). Recently, scientists performed experiments that strongly corroborated this hypothesis. They performed "in silico" mapping of the "adhesome," which is the molecular network dynamically assembled at focal adhesions upon integrin engagement (Zaidel-Bar et al. 2007). By examining existing protein interaction databases, the researchers learned that the adhesome contains more than 150 proteins. These proteins have a relatively small number of key sections in which a scaffolding molecule recruits both a signaling molecule and its downstream target (Figure 2).
By performing experiments that introduced subtle mutations in the integrin cytoplasmic domains, scientists collected more stringent evidence that integrins indeed carry out two distinct functions in cells. These mutations compromised selected signaling functions without impairing the receptor's overall adhesive function. Scientists have introduced knock-in mutations in both in the Drosophila βPS integrin gene (the orthologue of the ubiquitous mammalian β1 integrins) and in the mouse platelet-specific β3 integrin gene (Grinblat et al. 1994; Law et al. 1999). Both integrin mutants had functional defects that could be specifically ascribed to signaling function impairments.
Summary
References and Recommended Reading
Brembeck, F. H., Rosario, M. & Birchmeier, W. Balancing cell adhesion and Wnt signaling, the key role of beta-catenin. Current Opinion in Genetics and Development 16, 51–59 (2006).
Clevers, H. Wnt/beta-catenin signaling in development and disease. Cell 127, 469–480 (2006).
Frisch, S. M. & Screaton, R. A. Anoikis mechanisms. Current Opinion in Cell Biology 13, 555–562 (2001).
Grinblat, Y. et al. Functions of the cytoplasmic domain of the beta PS integrin subunit during Drosophila development. Development (Cambridge, England) 120, 91–102 (1994).
Gumbiner, B. M. Cell adhesion: The molecular basis of tissue architecture and morphogenesis. Cell 84, 345–357 (1996).
Gumbiner, B. M. Regulation of cadherin-mediated adhesion in morphogenesis. Nature Reviews 6, 622–634 (2005).
Johnson, M. S. et al. Integrins during evolution: Evolutionary trees and model organisms. Biochimica et Biophysica Acta 1788, 779–789 (2009).
Juliano, R. L. Signal transduction by cell adhesion receptors and the cytoskeleton: Functions of integrins, cadherins, selectins, and immunoglobulin-superfamily members. Annual Review of Pharmacology and Toxicology 42, 283–323 (2002).
Law, D. A. et al. Integrin cytoplasmic tyrosine motif is required for outside-in aIIbb3 signalling and platelet function. Nature 401, 808–811 (1999).
Legate, K. R., Wickstrom, S. A. & Fassler, R. Genetic and cell biological analysis of integrin outside-in signaling. Genes and Development 23, 397–418 (2009).
Maher, P. A. et al. Phosphotyrosine-containing proteins are concentrated in focal adhesions and intercellular junctions in normal cells. Proceedings of the National Academy of Sciences 82, 6576–6580 (1985).
Mousa, S. A. Cell adhesion molecules: Potential therapeutic and diagnostic implications. Molecular Biotechnology 38, 33–40 (2008).
Nelson, P. J. & Daniel, T. O. Emerging targets: Molecular mechanisms of cell contact-mediated growth control. Kidney International 61, S99–S105 (2002).
Parsons, J. T. et al. Focal adhesion kinase: Targeting adhesion signaling pathways for therapeutic intervention. Clinical Cancer Research 14, 627–632 (2008).
Takeichi, M. The cadherins: Cell-cell adhesion molecules controlling animal morphogenesis. Development (Cambridge, England) 102, 639–655 (1988).
Wang, N., Tytell, J. D., and Ingber, D. E. (2009). Mechanotransduction at a distance: Mechanically coupling the extracellular matrix with the nucleus. Nature Reviews 10, 75–82.
Wehrle-Haller, B. & Imhof, B. A. Integrin-dependent pathologies. Journal of Pathology 200, 481–487 (2003).
Zaidel-Bar, R. et al. Functional atlas of the integrin adhesome. Nature Cell Biology 9, 858–867 (2007).