« Prev Next »
Several elegant studies indicate that the small GTPase called Ras-related nuclear protein (Ran) is a central regulator of several cell cycle events. In the 1990s, scientists first discovered that Ran was an essential factor for protein import into the nucleus during interphase. In subsequent studies, they discovered that Ran had other functions in spindle assembly and nuclear envelope assembly during mitosis. While Ran modulates these vastly different cellular processes, it appears to use a gradient mechanism, wherein active Ran is more concentrated around its primary site of action and less concentrated elsewhere (Clarke & Zhang 2008).
How is the Ran Gradient Generated?
Ran binds either GTP or GDP and shuttles between the nucleus and cytoplasm. As a GTPase, Ran hydrolyzes bound GTP into GDP. The GTP-bound Ran (RanGTP) is considered active, while the GDP-bound Ran (RanGDP) is inactive. These alternate guanine-nucleotide states of Ran are regulated by two classes of partner proteins, which either promote the RanGTP or the RanGDP form. RanGEF (guanine-nucleotide exchange factor, RCC1) enhances the formation of RanGTP. The second group consists of RanGAP (GTPase-activating protein) and RanBPs (Ran-binding proteins: RanBP1 and RanBP2) which increases the GTPase activity of Ran and promotes the formation of RanGDP (Clarke & Zhang 2008, Stewart 2007, Xu & Massagué 2004).
The controlled cycling between active (RanGTP) and inactive (RanGDP) forms occurs in different regions of the cell, and enables the formation of the Ran gradient. In this exquisite strategy, RCC1 is imported to the nucleus and binds to chromatin, and RanGAP/RanBPs remains cytoplasmic. Consequently, RanGTP is prevalent in the nucleus, while RanGDP is concentrated in the cytoplasm, resulting in differential compartmentalization of RanGTP/RanGDP across the nuclear envelope during interphase (Figure 1; Clarke & Zhang 2008, Stewart 2007, Xu & Massagué 2004).
Compartmentalization of Ran Directs Nucleocytoplasmic Transport
To understand the import of nuclear proteins, pioneering researchers exploited a delicate experimental system in mammalian cells using a special chemical called digitonin. Digitonin selectively permeabilizes the plasma membrane of a cell without damaging the NE. Scientists then recreated an external cytoplasmic environment and introduced dyes that became fluorescent when they were imported into the NE. With this technique they could visualize the time-course of the nuclear import by fluorescence microscopy (Rabut et al. 2004, Yang & Musser 2006, Timney et al. 2006).
Soon after, other scientists identified the key proteins in the classical nuclear import pathway, which included Ran, importin α, importin β and NTF2. Importin α/β heterodimers acts as nuclear transport receptors and their interaction is disrupted by RanGTP. Importin β belongs to a larger family of transport factors, which includes both importins and exportins mediating either the nuclear import or export. In classical nucleocytoplasmic transport pathways, the cargo proteins typically contain a short protein sequence, either a nuclear localization signal (NLS) that directs proteins to the nucleus or a nuclear export signal (NES) that directs proteins to the cytoplasm. Importins form complexes with NLS cargoes and exportins form complexes with NES cargoes and translocate them across the NPCs (Clarke & Zhang 2008, Stewart 2007, Xu & Massagué, 2004, Strambio-De-Castillia et al. 2010).
How does Ran influence the import and export of proteins across the NE? Depending on its guanine-nucleotide bound state, Ran stimulates assembly or disassembly of cargo-receptor complexes. In the nucleus, where RanGTP is prevalent, NLS cargoes are released from the transport complexes, whereas NES cargoes are captured. NES cargoes are then transported across the NPCs together with RanGTP to the cytoplasm and released after the hydrolysis of RanGTP to RanGDP. How does Ran get back into the nucleus? Another protein that binds Ran, the nuclear transport factor-2 (NTF2) interacts preferentially with RanGDP and promotes rapid nuclear import of Ran, which guarantees an efficient recycling of Ran between nucleus and cytoplasm (Figure 1; Clarke & Zhang 2008, Stewart 2007, Xu & Massagué 2004, Strambio-De-Castillia et al. 2010).
The Ran Gradient also Controls Spindle Assembly
What has the Ran gradient got to do with spindle assembly? When dividing cells enter M-phase the NE disappears, and the microtubules reorganize into the spindle apparatus to separate the chromosomes. Surprisingly, scientists discovered that the Ran gradient persists during the M-phase and is important for spindle assembly. Similar to its function in nucleocytoplasmic transport, Ran directs spindle assembly to the correct chromosome position in two ways. First, RCC1 — the RanGEF — binds to DNA and histone proteins 2A and 2B (H2A, H2B), and recruits RanGTP to the location of spindle assembly on the chromosome. Second, these high concentrations of RanGTP around the spindle assembly regions then attract the importins and release the spindle assembly factors (SAFs), such as TPX2 and NuMA. SAFs are usually bound to nucleocytoplasmic transport receptors. TPX2 is a microtubule organizing factor that promotes spindle formation and is inhibited by the importin α/β heterodimer. When RanGTP binds importin α/β, TPX2 can then initiate microtubule formation. TPX2 also activates an important protein kinase — Aurora A — involved in cell division. Both functions of TPX2 contribute greatly to spindle assembly. Thus the Ran gradient around the spindle attachment point on the chromosome regulates spindle assembly in a spatiotemporal manner (Figure 2; Clarke & Zhang 2008, Fu et al. 2007).
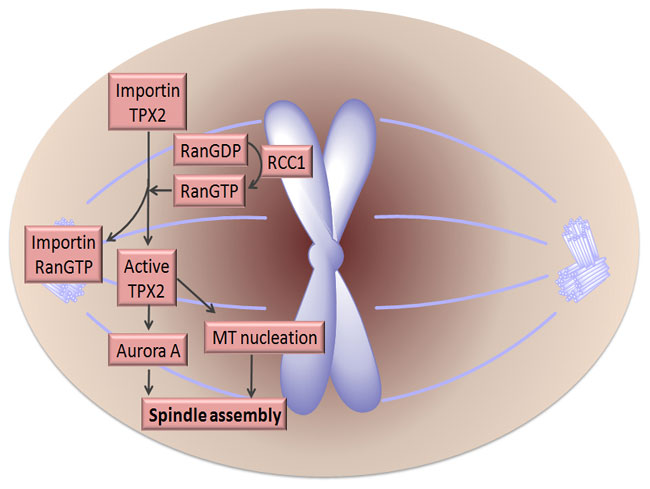

FRET: Advanced Technology to Visualize the Ran Gradient
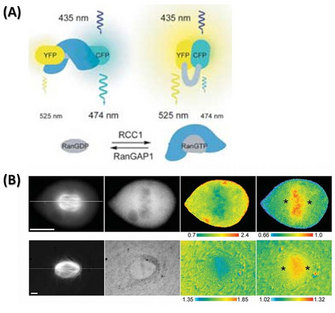

The same group also designed another FRET sensor to monitor the cargoes released by RanGTP from importin α/β. They used the importin β-binding (IBB) domain instead of RBD in this FRET sensor. Structurally, importin α exists in an auto-inhibitory state and can not bind to NLS cargoes. Only when importin β binds to the IBB domain of importin α can it then bind to NLS cargoes. Importin β preferentially binds to RanGTP and dissociates from importin α which in its unbound state releases the NLS cargoes. Therefore, the IBB FRET sensor could visualize the gradient of NLS cargos in mitosis. Using this probe, the chromosome in both Xenopus egg extracts and mitotic cells showed a high FRET signal, indicating free cargoes and high concentrations of RanGTP. This experiment gave a clear view of the Ran gradient and confirmed how it could regulate the downstream cargoes (Figure 3; Kalab et al. 2006).
Ran GTPase Cycle Participates in Nuclear Envelope Assembly
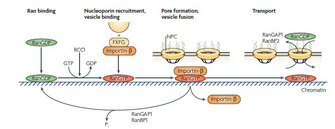

One road block to understanding the mechanism was that disruption of the Ran GTPase cycle has a vast impact on chromatin decondensation and the reestablishment of nucleocytoplasmic transport besides NE assembly. How could scientists know for sure whether Ran regulates NE formation directly, or indirectly, by affecting the other two processes? In 2000, Zhang & Clarke used a strategy with artificial beads to prove the direct function of Ran GTPase in NE assembly. The artificial beads are made of solid sepharose, and thus have the advantage to exclude the effects of chromatin while allowing NE assembly on the surface. Zhang & Clarke coated the artificial beads with Ran protein and added them to the Xenopus egg extracts and other cell-free systems. The membrane vesicles then accumulated around the beads and fused to form a continuous membrane with NPCs, indicating that Ran is directly involved in the NE formation in addition to its impact on the chromatin and the nucleocytoplasmic transport (Zhang & Clarke 2000, Clarke & Zhang 2008).
Now another big question is whether Ran exerts its function in the same way as in nucleocytoplasmic transport and spindle assembly. Does it reverse the inhibitory effect of importin α/β on important factors in nucelocytoplasmic transport? The prevailing view is that both importin α and importin β are key elements in the NE assembly, but their relationship with Ran is still not well defined (Figure 4).
Summary
The small GTPase, Ran, orchestrates multiple stages of the cell cycle, ranging from nucleocytoplasmic transport in interphase, to spindle assembly and nuclear envelope assembly during the M-phase. In the last decade, the research in this field has unified the mechanism by which Ran plays different roles in different cell cycle events. By the specific distribution of RanGTP/GDP across the NE, regulatory factors are released from inhibition of nucleocytoplasmic transport receptors in a controlled, spatiotemporal manner. Important questions still remain about Ran function during nuclear envelope assembly. Also, since Ran is such an important regulator, scientists still wonder, how does it coordinate with another family of master regulators, the CDKs (Cyclin-Dependent Kinases)?
References and Recommended Reading
Clarke, P. R. & Zhang, C. Spatial and temporal coordination of mitosis by Ran GTPase. Nature Reviews Molecular Cell Biology 9, 464–477 (2008).
Fu, J., Bian, M et al. Roles of Aurora Kinases in Mitosis and Tumorigenesis. Molecular Cancer Research 5, 1–10 (2007).
Güttinger, S., Laurell, E. et al. Orchestrating nuclear envelope disassembly and reassembly during mitosis. Nature Reviews Molecular Cell Biology 10, 178–191 (2009).
Kalab, P., Weis, K. et al. Visualization of a Ran-GTP gradient in interphase and mitotic Xenopus egg extracts. Science 295, 2452–2456 (2002).
Kalab, P., Pralle, A. et al. Analysis of a RanGTP-regulated gradient in mitotic somatic cells. Nature 440, 697–701 (2006).
Rabut, G., Doye, V. et al. Mapping the dynamic organization of the nuclear pore complex inside single living cells. Nature Cell Biology 6, 1114–1121 (2004).
Stewart. M. Molecular mechanism of the nuclear protein import cycle. Nature Reviews Molecular Cell Biology 8, 195–208 (2007).
Strambio-De-Castillia, C., Niepel, M. et al. The nuclear pore complex: bridging nuclear transport and gene regulation. Nature Reviews Molecular Cell Biology 11, 490–501 (2010).
Timney, B. L., Tetenbaum-Novatt, J. et al. Simple kinetic relationships and nonspecific competition govern nuclear import rates in vivo. Journal of Cell Biology 175, 579–593 (2006).
Xu, L. & Massagué. J. Nucleocytoplasmic shuttling of signal transducers. Nature Reviews Molecular Cell Biology 5, 209–219 (2004).
Yang, W. & Musser, S. M. Nuclear import time and transport efficiency depend on importin beta concentration. Journal of Cell Biology 174, 951–961 (2006).