Abstract
Despite its Earth-like size and source material1,2, Venus is extremely dry3,4, indicating near-total water loss to space by means of hydrogen outflow from an ancient, steam-dominated atmosphere5,6. Such hydrodynamic escape likely removed most of an initial Earth-like 3-km global equivalent layer (GEL) of water but cannot deplete the atmosphere to the observed 3-cm GEL because it shuts down below about 10–100 m GEL5,7. To complete Venus water loss, and to produce the observed bulk atmospheric enrichment in deuterium of about 120 times Earth8,9, nonthermal H escape mechanisms still operating today are required10,11. Early studies identified these as resonant charge exchange12,13,14, hot oxygen impact15,16 and ion outflow17,18, establishing a consensus view of H escape10,19 that has since received only minimal updates20. Here we show that this consensus omits the most important present-day H loss process, HCO+ dissociative recombination. This process nearly doubles the Venus H escape rate and, consequently, doubles the amount of present-day volcanic water outgassing and/or impactor infall required to maintain a steady-state atmospheric water abundance. These higher loss rates resolve long-standing difficulties in simultaneously explaining the measured abundance and isotope ratio of Venusian water21,22 and would enable faster desiccation in the wake of speculative late ocean scenarios23. Design limitations prevented past Venus missions from measuring both HCO+ and the escaping hydrogen produced by its recombination; future spacecraft measurements are imperative.
This is a preview of subscription content, access via your institution
Access options
Access Nature and 54 other Nature Portfolio journals
Get Nature+, our best-value online-access subscription
$29.99 / 30 days
cancel any time
Subscribe to this journal
Receive 51 print issues and online access
$199.00 per year
only $3.90 per issue
Buy this article
- Purchase on Springer Link
- Instant access to full article PDF
Prices may be subject to local taxes which are calculated during checkout
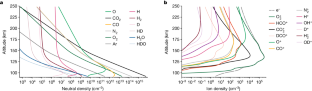


Similar content being viewed by others
Data availability
Tables containing all reactions used in the model, including their adopted rate coefficients and computed column rates, are provided in a supplementary PDF file accessible on the journal website. These rates are also accessible in the archived code repository listed below, which also includes our adopted photo cross-sections and all other source data used in our model. Model densities for all species, computed rates for reactions shown in Fig. 2, assumed temperature and escape probabilities and computed photo rates are provided in Excel format in the online version of the paper; this file also includes data for our illustrative water-inventory timelines. Source data are provided with this paper.
Code availability
All model code is available at github.com/emcangi/VenusPhotochemistry. The version of the model used to prepare the manuscript is archived on Zenodo at https://doi.org/10.5281/zenodo.10460004.
References
Izidoro, A. et al. Planetesimal rings as the cause of the Solar System’s planetary architecture. Nat. Astron. 6, 357–366 (2022).
Salvador, A. et al. Magma ocean, water, and the early atmosphere of Venus. Space Sci. Rev. 219, 51 (2023).
Moroz, V. I. et al. Spectrum of the Venus day sky. Nature 284, 243–244 (1980).
Marcq, E., Mills, F. P., Parkinson, C. D. & Vandaele, A. C. Composition and chemistry of the neutral atmosphere of Venus. Space Sci. Rev. 214, 10 (2018).
Kasting, J. F. & Pollack, J. B. Loss of water from Venus. I. Hydrodynamic escape of hydrogen. Icarus 53, 479–508 (1983).
Turbet, M. et al. Day–night cloud asymmetry prevents early oceans on Venus but not on Earth. Nature 598, 276–280 (2021).
Johnstone, C. P. Hydrodynamic escape of water vapor atmospheres near very active stars. Astrophys. J. 890, 79 (2020).
Donahue, T. M., Hoffman, J. H., Hodges, R. R. & Watson, A. J. Venus was wet: a measurement of the ratio of deuterium to hydrogen. Science 216, 630–633 (1982).
De Bergh, C. et al. Deuterium on Venus: observations from Earth. Science 251, 547–549 (1991).
Kumar, S., Hunten, D. M. & Pollack, J. B. Nonthermal escape of hydrogen and deuterium from Venus and implications for loss of water. Icarus 55, 369–389 (1983).
Donahue, T. M. New analysis of hydrogen and deuterium escape from Venus. Icarus 141, 226–235 (1999).
Stewart, A. I. F. in Second Arizona Conference on Planetary Atmospheres (1968).
Hodges, R. R. An exospheric perspective of isotopic fractionation of hydrogen on Venus. J. Geophys. Res. Planets 104, 8463–8471 (1999).
Chaufray, J.-Y., Bertaux, J.-L., Quémerais, E., Villard, E. & Leblanc, F. Hydrogen density in the dayside Venusian exosphere derived from Lyman-α observations by SPICAV on Venus Express. Icarus 217, 767–778 (2012).
McElroy, M. B., Prather, M. J. & Rodriguez, J. M. Escape of hydrogen from Venus. Science 215, 1614–1615 (1982).
Gu, H., Cui, J., Niu, D. & Yu, J. Hydrogen and helium escape on Venus via energy transfer from hot oxygen atoms. Mon. Not. R. Astron. Soc. 501, 2394–2402 (2021).
Hartle, R. E. & Grebowsky, J. M. Light ion flow in the nightside ionosphere of Venus. J. Geophys. Res. Planets 98, 7437–7445 (1993).
Persson, M. et al. H+/O+ escape rate ratio in the Venus magnetotail and its dependence on the solar cycle. Geophys. Res. Lett. 45, 10805–10811 (2018).
Lammer, H. et al. Loss of hydrogen and oxygen from the upper atmosphere of Venus. Planet. Space Sci. 54, 1445–1456 (2006).
Gillmann, C. et al. The long-term evolution of the atmosphere of Venus: processes and feedback mechanisms. Space Sci. Rev. 218, 56 (2022).
Grinspoon, D. H. Implications of the high D/H ratio for the sources of water in Venus’ atmosphere. Nature 363, 428–431 (1993).
Avice, G. et al. Noble gases and stable isotopes track the origin and early evolution of the Venus atmosphere. Space Sci. Rev. 218, 60 (2022).
Way, M. J. & Del Genio, A. D. Venusian habitable climate scenarios: modeling Venus through time and applications to slowly rotating Venus-like exoplanets. J. Geophys. Res. Planets 125, e06276 (2020).
Chaffin, M. S., Deighan, J., Schneider, N. M. & Stewart, A. I. F. Elevated atmospheric escape of atomic hydrogen from Mars induced by high-altitude water. Nat. Geosci. 10, 174–178 (2017).
Cangi, E. M., Chaffin, M. S. & Deighan, J. Higher Martian atmospheric temperatures at all altitudes increase the D/H fractionation factor and water loss. J. Geophys. Res. Planets 125, e06626 (2020).
Cangi, E., Chaffin, M., Yelle, R., Gregory, B. & Deighan, J. Fully coupled photochemistry of the deuterated ionosphere of Mars and its effects on escape of H and D. J. Geophys. Res. Planets 128, e2022JE007713 (2023).
Yung, Y. L. & Demore, W. B. Photochemistry of the stratosphere of Venus: implications for atmospheric evolution. Icarus 51, 199–247 (1982).
Fox, J. L. & Sung, K. Y. Solar activity variations of the Venus thermosphere/ionosphere. J. Geophys. Res. Space Phys. 106, 21305–21335 (2001).
Krasnopolsky, V. A. A photochemical model for the Venus atmosphere at 47–112 km. Icarus 218, 230–246 (2012).
Fedorova, A. et al. HDO and H2O vertical distributions and isotopic ratio in the Venus mesosphere by Solar Occultation at Infrared spectrometer on board Venus Express. J. Geophys. Res. Planets 113, E00B22 (2008).
Paxton, L. J., Anderson Jr, D. E. & Stewart, A. I. F. Analysis of Pioneer Venus Orbiter ultraviolet spectrometer Lyman α data from near the subsolar region. J. Geophys. Res. Space Phys. 93, 1766–1772 (1988).
Fox, J. L. The post-terminator ionosphere of Venus. Icarus 216, 625–639 (2011).
Brinton, H. C. et al. Venus nighttime hydrogen bulge. Geophys. Res. Lett. 7, 865–868 (1980).
Martinez, A. et al. Exploring the variability of the Venusian thermosphere with the IPSL Venus GCM. Icarus 389, 115272 (2023).
Navarro, T. et al. Venus’ upper atmosphere revealed by a GCM: I. Structure and variability of the circulation. Icarus 366, 114400 (2021).
Fox, J. L. The chemistry of protonated species in the Martian ionosphere. Icarus 252, 366–392 (2015).
Taylor, H. A., Brinton, H. C., Wagner, T. C. G., Blackwell, B. H. & Cordier, G. R. Bennett ion mass spectrometers on the Pioneer Venus Bus and Orbiter. IEEE Tran. Geosci. Remote Sens. 18, 44–49 (1980).
Miller, K. L., Knudsen, W. C. & Spenner, K. The dayside Venus ionosphere: I. Pioneer-Venus retarding potential analyzer experimental observations. Icarus 57, 386–409 (1984).
Barabash, S. et al. The Analyser of Space Plasmas and Energetic Atoms (ASPERA-4) for the Venus Express mission. Planet. Space Sci. 55, 1772–1792 (2007).
Bertaux, J. L. & Clarke, J. T. Deuterium content of the Venus atmosphere. Nature 338, 567–568 (1989).
Donahue, T. M. Deuterium on Venus. Nature 340, 513–514 (1989).
Liang, M.-C. & Yung, Y. L. Modeling the distribution of H2O and HDO in the upper atmosphere of Venus. J. Geophys. Res. Planets 114, E00B28 (2009).
Parkinson, C. D. et al. Photochemical control of the distribution of Venusian water. Planet. Space Sci. 113, 226–236 (2015).
Widemann, T. et al. Venus evolution through time: key science questions, selected mission concepts and future investigations. Space Sci. Rev. 219, 56 (2023).
McClintock, W. E. et al. The Imaging Ultraviolet Spectrograph (IUVS) for the MAVEN Mission. Space Sci. Rev. 195, 75–124 (2015).
Bertaux, J. L., Goutail, F., Dimarellis, E., Kockarts, G. & van Ransbeeck, E. First optical detection of atomic deuterium in the upper atmosphere from Spacelab 1. Nature 309, 771–773 (1984).
Gregory, B. S., Elliott, R. D., Deighan, J., Gröller, H. & Chaffin, M. S. HCO+ dissociative recombination: a significant driver of nonthermal hydrogen loss at Mars. J. Geophys. Res. Planets 128, e2022JE007576 (2023).
Barth, C. A., Pearce, J. B., Kelly, K. K., Wallace, L. & Fastie, W. G. Ultraviolet emissions observed near Venus from Mariner V. Science 158, 1675–1678 (1967).
Anderson, D. E. The Mariner 5 ultraviolet photometer experiment: analysis of hydrogen Lyman alpha data. J. Geophys. Res. 81, 1213–1216 (1976).
Takacs, P., Broadfoot, A., Smith, G. & Kumar, S. Mariner 10 observations of hydrogen Lyman alpha emission from the Venus exosphere: evidence of complex structure. Planet. Space Sci. 28, 687–701 (1980).
von Zahn, U., Kumar, S., Niemann, H. & Prinn, R. in Venus (eds Hunten D. M., Colin, L., Donahue, T. M. & Moroz, V. I.) 299–430 (Univ. Arizona Press, 1983).
Hunten, D. M. The escape of light gases from planetary atmospheres. J. Atmos. Sci. 30, 1481–1494 (1973).
Krissansen-Totton, J., Fortney, J. J. & Nimmo, F. Was Venus ever habitable? Constraints from a coupled interior–atmosphere–redox evolution model. Planet. Sci. J. 2, 216 (2021).
Warren, A. O. & Kite, E. S. Narrow range of early habitable Venus scenarios permitted by modeling of oxygen loss and radiogenic argon degassing. Proc. Natl Acad. Sci. 120, e2209751120 (2023).
Chassefière, E. Hydrodynamic escape of hydrogen from a hot water-rich atmosphere: the case of Venus. J. Geophys. Res. 101, 26039–26056 (1996).
Chassefière, E. Loss of water on the young Venus: the effect of a strong primitive solar wind. Icarus 126, 229–232 (1997).
Way, M. J. et al. Was Venus the first habitable world of our solar system? Geophys. Res. Lett. 43, 8376–8383 (2016).
Gillmann, C. et al. Dry late accretion inferred from Venus’s coupled atmosphere and internal evolution. Nat. Geosci. 13, 265–269 (2020).
Selsis, F., Leconte, J., Turbet, M., Chaverot, G. & Bolmont, É. A cool runaway greenhouse without surface magma ocean. Nature 620, 287–291 (2023).
Fox, J. L. & Bougher, S. W. Structure, luminosity, and dynamics of the Venus thermosphere. Space Sci. Rev. 55, 357–489 (1991).
Hodges Jr, R. R. Collision cross sections and diffusion parameters for H and D in atomic oxygen. J. Geophys. Res. 98, 3799–3805 (1993).
Shizgal, B. D. Escape of H and D from Mars and Venus by energization with hot oxygen. J. Geophys. Res. 104, 14833–14846 (1999).
Yang, J., Boué, G., Fabrycky, D. C. & Abbot, D. S. Strong dependence of the inner edge of the habitable zone on planetary rotation rate. Astrophys. J. 787, L2 (2014).
Herrick, R. R. & Hensley, S. Surface changes observed on a Venusian volcano during the Magellan mission. Science 379, 1205–1208 (2023).
Rolf, T. et al. Dynamics and evolution of Venus’ mantle through time. Space Sci. Rev. 218, 70 (2022).
Hedin, A. E., Niemann, H. B., Kasprzak, W. T. & Seiff, A. Global empirical model of the Venus thermosphere. J. Geophys. Res. 88, 73–84 (1983).
Bertaux, J.-L. et al. SPICAV on Venus Express: three spectrometers to study the global structure and composition of the Venus atmosphere. Planet. Space Sci. 55, 1673–1700 (2007).
Hagemann, R., Nief, G. & Roth, E. Absolute isotopic scale for deuterium analysis of natural waters. Absolute D/H ratio for SMOW. Tellus 22, 712–715 (1970).
Lillis, R. et al. Photochemical escape of oxygen from Mars: first results from MAVEN in situ data. J. Geophys. Res. Space Phys. 122, 3815–3836 (2017).
Rosati, R. E., Skrzypkowski, M. P., Johnsen, R. & Golde, M. F. Yield of excited CO molecules from dissociative recombination of HCO+ and HOC+ ions with electrons. J. Chem. Phys. 126, 154302–154302 (2007).
Miller, K. L., Knudsen, C. W., Spenner, K., Whitten, R. C. & Novak, V. Solar zenith angle dependence of ionospheric ion and electron temperatures and density on Venus. J. Geophys. Res. Space Phys. 85, 7759–7764 (1980).
Brace, L. H. et al. The dynamic behavior of the Venus ionosphere in response to solar wind interactions. J. Geophys. Res. 85, 7663–7678 (1980).
Kasprzak, W. T. et al. in Venus II: Geology, Geophysics, Atmosphere, and Solar Wind Environment (eds Bougher, S. W. et al.) 225–258 (Univ. Arizona Press, 1997).
Niemann, H. B., Kasprzak, W. T., Hedin, A. E., Hunten, D. M. & Spencer, N. W. Mass spectrometric measurements of the neutral gas composition of the thermosphere and exosphere of Venus. J. Geophys. Res. Space Res. 85, 7817–7827 (1980).
Fox, J. L. & Kliore, A. J. in Venus II: Geology, Geophysics, Atmosphere, and Solar Wind Environment (eds Bougher, S. W. et al.) 161–188 (Univ. Arizona Press, 1997).
Grebowsky, J. M., Kasprzak, W. T., Hartle, R. E. & Donahue, T. M. A new look at Venus’ thermosphere H distribution. Adv. Space Res. 17, 191–195 (1996).
Donahue, T. M., Grinspoon, D. H., Hartle, R. E. & Hodges, R. R. Jr. in Venus II: Geology, Geophysics, Atmosphere, and Solar Wind Environment (eds Bougher, S. W. et al.) 385–414 (Univ. Arizona Press, 1997).
Stolzenbach, A., Lefèvre, F., Lebonnois, S. & Määttänen, A. Three-dimensional modeling of Venus photochemistry and clouds. Icarus 395, 115447 (2023).
Dickinson, R. E. & Ridley, E. C. Venus mesosphere and thermosphere temperature structure: II. Day-night variations. Icarus 30, 163–178 (1977).
Seiff, A. Dynamical implications of the observed thermal contrasts in Venus’ upper atmosphere. Icarus 51, 574–592 (1982).
Garvin, J. B. et al. Revealing the mysteries of Venus: the DAVINCI mission. Planet. Sci. J. 3, 117 (2022).
Smrekar, S. E. et al. VERITAS (Venus Emissivity, Radio Science, InSAR, Topography, and Spectroscopy): a selected discovery mission in 53rd Lunar and Planetary Science Conference. LPI contribution no. 2678, id. 1122 (2022).
Helbert, J. et al. The VenSpec suite on the ESA Envision mission – a holistic investigation of the coupled surface atmosphere system of Venus in 16th Europlanet Science Congress, id. EPSC2022-374 (2022).
Acknowledgements
M.S.C., E.M.C., B.S.G. and R.D.E. were supported by NASA Solar System Workings grant 80NSSC19K0164 and Planetary Science Early Career Award grant 80NSSC20K1081. E.M.C. was also supported by NASA FINESST award 80NSSC22K1326. M.S.C. and E.M.C. thank M. Landis for helpful discussions about water delivery.
Author information
Authors and Affiliations
Contributions
M.S.C. oversaw the study, performed final model calculations and the photochemical equilibrium calculation and wrote the initial text of the paper. E.M.C. developed the H-bearing and D-bearing photochemical model and nonthermal escape calculation originally used at Mars with a reaction network provided by R.V.Y. and performed initial model calculations for Venus. B.S.G. developed and ran the Monte Carlo model to generate escape probability curves. R.D.E. initially developed the Monte Carlo escape model with support from J.D. and H.G. H.G. performed pilot studies of HCO+-driven loss in the Mars atmosphere. All authors contributed to the interpretation and presentation of model results.
Corresponding author
Ethics declarations
Competing interests
The authors declare no competing interests.
Peer review
Peer review information
Nature thanks David Grinspoon and the other, anonymous, reviewer(s) for their contribution to the peer review of this work. Peer reviewer reports are available.
Additional information
Publisher’s note Springer Nature remains neutral with regard to jurisdictional claims in published maps and institutional affiliations.
Extended data figures and tables
Extended Data Fig. 1 Model densities for all species.
The six panels function only to separate species for clarity.
Extended Data Fig. 2 Key photochemical model inputs.
a, Temperature profiles for neutrals, ions and electrons adapted from the inputs in ref. 28. b, Adopted eddy diffusion profile and molecular diffusion coefficients for H and O atoms.
Extended Data Fig. 3 Implications of HCO+-driven loss for Venus ocean scenarios.
a, Escaping H production rates for the two most important processes in our model. b, Schematic water loss history of Venus.
Supplementary information
Supplementary Information
This file contains Supplementary Methods and Supplementary Tables. Merged PDF containing tables of reactions used in the model, assumed reaction rate coefficients and computed equilibrium model column rates.
Source data
Rights and permissions
Springer Nature or its licensor (e.g. a society or other partner) holds exclusive rights to this article under a publishing agreement with the author(s) or other rightsholder(s); author self-archiving of the accepted manuscript version of this article is solely governed by the terms of such publishing agreement and applicable law.
About this article
Cite this article
Chaffin, M.S., Cangi, E.M., Gregory, B.S. et al. Venus water loss is dominated by HCO+ dissociative recombination. Nature 629, 307–310 (2024). https://doi.org/10.1038/s41586-024-07261-y
Received:
Accepted:
Published:
Issue Date:
DOI: https://doi.org/10.1038/s41586-024-07261-y
Comments
By submitting a comment you agree to abide by our Terms and Community Guidelines. If you find something abusive or that does not comply with our terms or guidelines please flag it as inappropriate.