« Prev Next »
Introduction and Historical Perspective
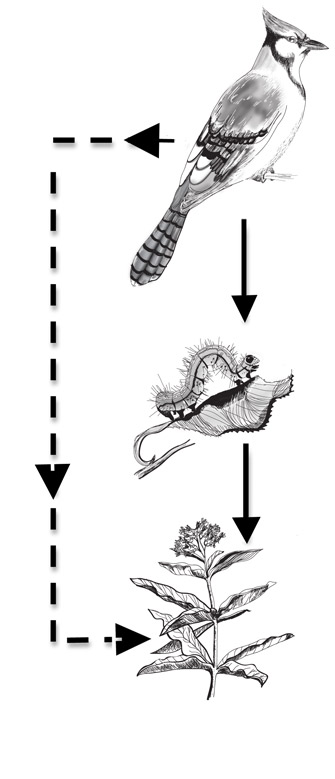

Historically, ecologists focused their attention on understanding direct effects such as competition, herbivory, and predation (e.g., Park 1948, Huffaker 1958, Connell 1961). Such direct effects were believed to be the forces that primarily shaped population and community structure. Competition was thought to be particularly important as species partitioned their niches, ecologically and evolutionarily, to lessen the impact of interspecific competition (MacArthur 1958, Hutchinson 1959). Competition was also at the forefront of experimental ecology, with the work of ecologists such as Thomas Park (1954) and Joe Connell (1961) significantly influencing how we viewed interspecific interactions and how we went about studying them. Interestingly, Park's work on competition also illustrated the importance of indirect effects by demonstrating that the outcome of competitive interactions between Tribolium castaneum and Tribolium confusum changed when the beetles were infected with the parasite Adelina (Park 1948). Park's experiments also showed that the outcome of interspecific interactions can be changed by variation in abiotic environmental factors (Park 1954). In general, however, indirect effects were thought to be relatively unimportant in shaping community structure (Vandermeer 1969) and received little attention. Because of their complexity, indirect effects may be more difficult to detect and quantify than direct effects, and this may have contributed to the underestimation of their role in communities (Menge 1995, Strong 1997).
In spite of a lack of experimental and theoretical treatment by ecologists, evidence that indirect effects can be strong, and important, had been present for many years. Extensive use of biological control testifies to the potential strength of indirect effects, as crop yield is increased by trophic cascades initiated by introduced natural enemies or the populations of naturally occurring predators and parasites are augmented. Hairston et al. (1960) in their seminal "green world" paper brought this concept from an agricultural setting to a natural one by suggesting that the world is green because predators and parasites control herbivore densities, allowing plants to proliferate (often referred to as the HSS hypothesis). Indeed, studies on trophic cascades began to proliferate, and they have received more theoretical and empirical treatment than any other indirect effect pathway (reviewed in Brett & Goldman 1996, Schmitz et al. 2000, Halaj & Wise 2001). Studies demonstrating the importance of other indirect effects proliferated as well, and it became clear that indirect effects can be important determinants of community structure (e.g., Strauss 1991a, b; Wootton 1993, 1994).
With the understanding that indirect effects can be important came the desire to understand the relative strengths of direct and indirect effects in natural ecosystems. In a review of experimental studies, Schoener (1993) reported that only about one quarter of all community change reported resulted from indirect effects while the remainder resulted from direct effects. This review seemed to support the earlier view that relative to direct effects, indirect effects were not very important. Menge (1995), however, reported that, on average, 40–45% of all community change resulted from indirect effects and that in individual studies the percentage could be as high as 65%. Thus, it seemed that the relative roles of these factors varied among studies and that under certain circumstances, indirect effects could be as strong as direct effects.
Understanding the factors that shift the relative importance of direct and indirect effects continues to be a focal point of studies of species interactions. Fretwell and Oksanen and colleagues (Fretwell 1977, Oksanen et al. 1981) applied this concept to the earlier HSS model. Oksanen et al. suggested that the scenario proposed by Hairston et al. was one possibility, but the indirect effects of natural enemies on plants were not always strong. They suggested that the productivity of the ecosystem determined the relative strengths of various direct and indirect effects among species. For example, in ecosystems with very low productivity, plants would be limited by competition for resources and would not produce enough biomass to support herbivores. As productivity increased, herbivore populations could be supported and plant populations would now be limited by herbivory. With further increases in productivity, natural enemies could now be supported and they would limit herbivore population sizes, resulting in a positive indirect effect on plants. If productivity is sufficient for supporting a fourth trophic level, these secondary enemies would have a positive indirect effect on herbivores and a negative indirect effect on plants. Menge & Sutherland (1976) described how similar scenarios could play out with varying levels of environmental stress.
The HSS, Oksanen-Fretwell, and Menge-Sutherland hypotheses not only addressed the issue of the relative importance of direct and indirect effects in communities but also sparked an associated debate regarding the importance of top-down versus bottom-up factors. Hunter & Price (1992) established a theoretical framework in which environmental factors set the stage upon which interspecific interactions act. Their model integrated direct and indirect effects, top-down and bottom-up factors, and environmental heterogeneity and established the paradigm for many future studies, including our own, some of which we discuss below.
Lessons from the Marsh
Salt marshes provide excellent opportunities for examining the effects of environmental heterogeneity on direct and indirect interactions. Salt marshes are spatially and temporally variable in a number of abiotic factors, including nutrient levels, which can drive productivity, and salinity, which can cause plant stress (Adam 1990). Additionally, communities in salt marshes tend to be simpler than in many other habitats, which facilitates the elucidation of direct and indirect interaction pathways, and makes the experimental manipulation of these pathways and species in the field logistically feasible (Denno et al. 2002, Moon & Stiling 2004). Studying interspecific interactions in the salt marsh has allowed for explicit testing of many of the ideas proposed in the HSS, Oksanen-Fretwell, Menge-Sutherland, and Hunter & Price models and has revealed how consideration of both direct and indirect effects in the context of environmental heterogeneity is essential for understanding community dynamics.
Many of our studies have focused on the arthropod community associated with the sea oxeye daisy, Borrichia frutescens (Figures 2 & 3), which is a perennial composite that inhabits marsh zones just shoreward of the intertidal zone along Florida's Atlantic and Gulf coasts. Many studies have shown that herbivorous insect densities are related to nutrient availability (e.g., Mattson 1980, Dixon 1985, Waring & Cobb 1992). This has been shown to be true for the herbivores of B. frutescens as well. A series of field experiments showed that increasing nitrogen availability, by fertilizing plants in the field, resulted in positive direct effects on densities of the phloem-feeding homopteran Pissonotus quadripustulatus, the gall midge Asphondylia borrichiae, and the lepidopteran stem borer Argyresthia sp. (Figure 2, Moon et al. 2000; Moon & Stiling 2000, 2002b, c, 2004). Nitrogen availability was also decreased by adding labile carbon in the form of sugar and densities of insect herbivores tracked these decreases as well (Stiling & Moon 2005). Although the magnitude of change differed among herbivore species, all responded similarly to the increases and decreases in nutrient availability because of the changes in host plant nitrogen content occurring at the same time.
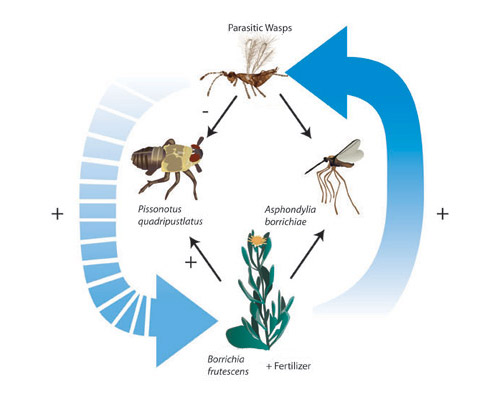

Changes in soil salinity levels also alter plant quality and morphology, which results in direct effects on herbivores. In the case of salinity, unlike nitrogen, effects varied among herbivore species. Increasing levels of soil pore water salinity by experimentally adding salt resulted in higher densities of the planthopper P. quadripustulatus (Moon & Stiling 2000) but resulted in lower densities of gall midge A. borrichiae (Figure 3, Moon & Stiling 2002a). For P. quadripustulatus, the increase may have resulted from mobilization of amino acids to achieve a more favorable osmotic balance (Cavalieri & Huang 1979, White 1984). In contrast, the gall midge A. borrichiae may have been affected by lower moisture content and a toughening of the plant tissues that occurs in more saline environments (Moon & Stiling 2002a).
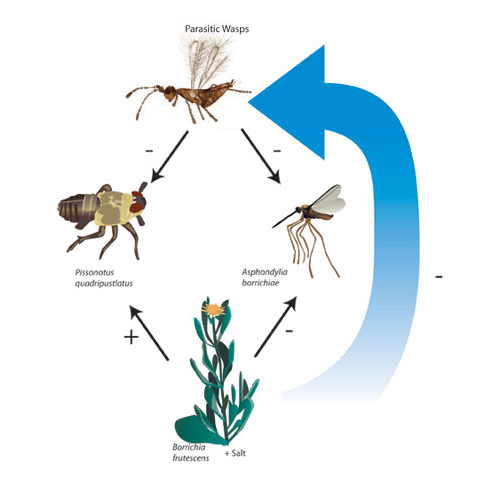

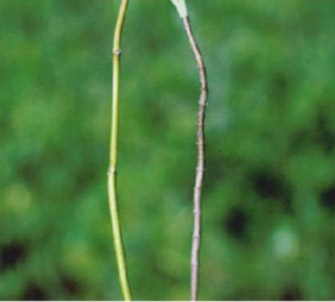

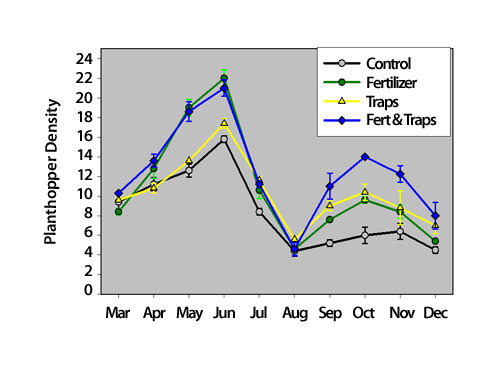
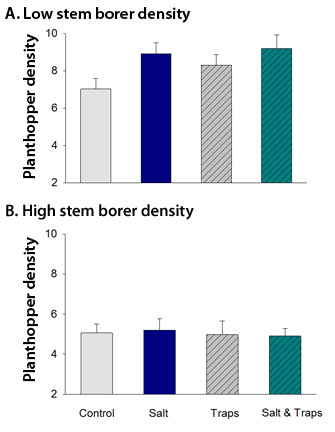

As suggested by theoretical models such as those of Oksanen et al. (1981), Menge & Sutherland (1976), and Hunter & Price (1992), abiotic heterogeneity in the salt marsh can affect the relative importance of direct and indirect top-down and bottom-up interactions. Alberti et al. (2010) recently showed similar strong effects of the abiotic environment on trophic interactions in a salt marsh in Argentina. Salt marshes are not the only ecosystems in which we find abiotically-mediated direct and indirect effects. They are widespread in ecosystems ranging from the Barents Sea in the Arctic Ocean (Ciannelli et al. 2007) to Africa's Serengeti (Sinclair et al. 2007). Understanding how abiotic factors mediate direct and indirect interactions is not only important theoretically but practically as well. Anthropogenic modification of the abiotic environment through pollution, eutrophication, and global climate change continues to increase. Understanding and predicting the impact of these anthropogenic effects on interactions between species will be one of the most important challenges community ecologists face into the future.
References and Recommended Reading
Adam, P. Saltmarsh Ecology. Cambridge, UK: Cambridge University Press, 1990.
Alberti, J. et al. Abiotic stress mediates top-down and bottom-up control in a Southwestern Atlantic salt marsh. Oecologia 163, 181–191 (2010).
Bender, E. A. et al. Perturbation experiments in community ecology: theory and practice. Ecology 65, 1–13 (1984).
Brett, M. T. & Goldman, C. R. A meta-analysis of the freshwater trophic cascade. Proceedings of the National Academy of Sciences 93, 7723–7726 (1996).
Byers, J. E. et al. Variable direct and indirect effects of a habitat modifying invasive species on mortality of native fauna. Ecology 91, 1787–1798 (2010).
Cavalieri, A. J. & Huang, A. H. C. Evaluation of proline accumulation in the adaptation of diverse species of salt marsh halophytes to the saline environment. American Journal of Botany 66, 307–312 (1979).
Ciannelli, L. et al. Spatial anatomy of species survival: effects of predation and climate-driven environmental variability. Ecology 88, 635–646 (2007).
Connell, J. H. The influence of interspecific competition and other factors on the distribution of the barnacle Cthamalus stellatus. Ecology 42, 710–723 (1961).
Denno, R. E. et al. Bottom-up forces mediate natural enemy impact in a phytophagous insect community. Ecology 83, 1443–1458 (2002).
Dixon, A. F. G. Aphid Ecology. Glasgow, Scotland: Blackie, 1985.
Fretwell, S. The regulation of plant communities by the food chains exploiting them. Perspectives in Biology and Medicine 20, 169–185 (1977).
Hairston, N. G. et al. Community structure, population control, and competition. American Naturalist 44, 421–425 (1960).
Halaj, J. & Wise, D. H. Terrestrial trophic cascades: how much do they trickle?American Naturalist 157, 262–281 (2001).
Huffaker, C. B. Experimental studies on predation: dispersion factors and predator-prey oscillations. Hilgardia 27, 343–383 (1958).
Hunter, M. D. & Price, P. W. Playing chutes and ladders: heterogeneity and the relative roles of bottom-up and top-down forces in natural communities. Ecology 73, 724–732 (1992).
Hutchinson, G.E. Homage to Santa Rosalia or why are there so many kinds of animals?American Naturalist 93, 145-159 (1959).
MacArthur, R. Population ecology of some warblers of northeastern coniferous forests. Ecology 39, 599–619 (1958).
Mattson, W. J. Herbivory in relation to plant nitrogen content. Annual Review of Ecology and Systematics 65, 119–161 (1980).
Menge, B. A. Indirect effects in marine rocky intertidal interaction webs: patterns and importance. Ecological Monographs 65, 21–74 (1995).
Menge, B. A. Detection of direct versus indirect effects: were experiments long enough? American Naturalist 149, 801 (1997).
Menge, B. A. & Sutherland, J. P. Species diversity gradients: synthesis of the roles of predation, competition and temporal heterogeneity. American Naturalist 110, 351–369 (1976).
Moon, D. C. et al. The effects of abiotically induced changes in host plant quality (and morphology) on a salt marsh planthopper and its parasitoid. Ecological Entomology 25, 325–331 (2000).
Moon, D.C. & Stiling, P. Relative importance of abiotically-induced direct and indirect effects on a salt-marsh herbivore. Ecology 81, 470–481 (2000).
———. The effects of salinity and nutrients on a tritrophic salt marsh system. Ecology 83, 2465–2476 (2002a).
———. The Effects of herbivore feeding mode on top-down and bottom-up effects in a salt marsh ecosystem. Oecologia 133, 243–253 (2002b).
———. Top-down, bottom-up, or side to side? Within-trophic-level interactions modify trophic dynamics of a salt marsh herbivore. Oikos 98, 480–490 (2002c).
———. The relative importance of top-down and bottom-up forces in coastal versus upland tritrophic complexes. Ecology 85, 2709–2716 (2004).
———. Effects of nutrients and parasitism suppressed by within-trophic-level interactions. Ecological Entomology 30, 642–649 (2005).
———. Trade-off in oviposition strategy: choosing poor quality host plants reduces mortality from natural enemies for a salt marsh planthopper. Ecological Entomology 31, 1–6 (2006).
Oksanen, L. et al. Exploitation ecosystems in gradients of primary productivity. American Naturalist 118, 240–261 (1981).
Park, T. Experimental studies of interspecies competition. I. Competition between populations of the flour beetles Tribolium confusum Duvall and Tribolium castaneum Herbst. Ecological Monographs 18, 267–307 (1948).
———. Experimental studies of interspecies competition. II. Temperature, humidity, and competition in two species of Tribolium. Physiological Zoology 27, 177–238 (1954).
Schmitz, O. J. et al. Trophic cascades in terrestrial systems: a review of the effects of carnivore removals on plants. American Naturalist 155, 141–153 (2000).
Schoener, T. "On the relative importance of direct versus indirect effects in ecological communities." In Mutualism and Community Organization: Behavioral, Theoretical, and Food Web Approaches, eds. H. Kawanabe, J. E. Cohen, & K. Wasaki (Oxford: Oxford University Press, 1993): 365–411.
Sinclair, A. R. E. et al. Long-Term Ecosystem Dynamics in the Serengeti: Lessons for Conservation. Conservation Biology 21, 580–590 (2007).
Stiling, P. & Moon, D. C. Are trophodynamic models worth their salt? The relative roles of top-down and bottom-up forces along a salinity gradient in a Florida salt marsh. Ecology 86, 1730–1736 (2005).
———. Quality or quantity: the direct and indirect effects of host plants on herbivores and their natural enemies. Oecologia 142, 413–420 (2005).
Strauss, S. Y. Indirect Effects in Community Ecology Their Definition Study and Importance. Trends in Ecology and Evolution 6, 206–210 (1991).
———. Direct, Indirect, and Cumulative Effects of 3 Native Herbivores on a Shared Host Plant. Ecology 72, 543–558 (1991).
Strong, D. R. Quick indirect interactions in intertidal food webs. Trends in Ecology and Evolution 12, 173–174 (1997).
Vandermeer, J. H. The competitive structure of communities: an experimental approach with Protozoa. Ecology 50, 362–371 (1969).
Waring, G. L. & Cobb, N. S. The impact of plant stress on herbivore population dynamics. In Insect-Plant Interactions, 4th vol., ed. E. Bernays (Boca Raton: CRC Press, 1992): 168–226.
White, T. C. R. The availability of invertebrate herbivores in relation to the availability of nitrogen in stressed food plants. Oecologia 63, 71–86 (1984).
Wootton, J. T. Indirect effects and habitat use in an intertidal community: interaction chains and interaction modifications. American Naturalist 141, 71–79 (1993).
Wootton, J. T. The nature and consequences of indirect effects in ecological communities. Annual Review of Ecology and Systematics 25, 443–466 (1994).
Younginger, B. et al. Interactive effects of mycorrhizal fungi, salt stress, and exploitative competition on the herbivores of Baccharis halimifolia. Ecological Entomology 34, 580–587 (2009).