Abstract
Resistance to phosphine is widely reported in several stored product insect pests globally. However, knowledge of its prevalence and the association of antioxidant enzymes with phosphine resistance is limited. Herein, we assessed the levels of phosphine susceptibility and estimated the antioxidant enzyme activities viz., superoxide dismutase (SOD), peroxidase (POX), and catalase (CAT) in selected Indian populations of red flour beetle Tribolium castaneum (Herbst). Dose–response probit assays revealed that the LC50 values ranged from 0.038 to 1.277 mg L−1 showing 2.11 to 70.94-fold resistance to phosphine compared to susceptible check. Activities of antioxidant enzymes varied significantly between the T. castaneum populations following phosphine exposure. The magnitude of SOD activity ranged from 8.77 to18.82 U mg−1 protein, while, the activities of POX and CAT varied between 52.42 and 408.32 and 61.11 to 247.49 µM H2O2 reduced min−1 mg−1 of protein, respectively. The correlation analysis revealed a significant positive association of SOD (r = 0.89) and POX (r = 0.98) with increased resistance ratio, while the CAT (r = − 0.98) is negatively linked with resistance to phosphine. A principal component analysis identified phosphine resistance was closely associated with POX and SOD activities but was unrelated to the CAT activity. Our results throw light on the varied association of antioxidant enzyme activities in response to phosphine fumigation in field populations of T. castaneum. Further studies on the biochemical and molecular basis of phosphine stress in insects may help to devise suitable strategies to safeguard storage commodities and ensure a sustainable environment.
Similar content being viewed by others
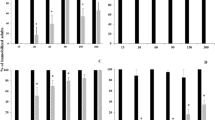
Introduction
Food grains are more prone to biotic stresses in stored conditions. Insects are one of the prime causes of deterioration in quality and quantity in post-harvest storage. The damage losses are estimated to account for approximately 5–10% and 20–30% in temperate and tropical countries worldwide, respectively1.The red flour beetle, Tribolium castaneum (Herbst) (Coleoptera,Tenebrionidae), is an important secondary pest of stored agricultural commodities wrecking damage to flour, cereal, beans, and nuts2. Apart from direct losses, this beetle secrets benzoquinones (viz., a carcinogenic substance with persistent and objectionable odour) that influence characteristic colour change of the flour and cause severe health risks to human consumption3. Thus, T. castaneum exaggerates both the quality and quantity of food products.
Phosphine is an eco-friendly and economically affordable fumigant used worldwide for management of stored product insect pests. However, prolonged use of the fumigant and adoption of inadequate fumigation practices have resulted in development of resistance to this fumigant by several stored product insect pests4,5. Non availability of suitable alternative fumigant makes phosphine inevitable in control of storage pests in bulk grain storages.
Studies have documented strong resistance to phosphine in T. castaneum populations from USA6,7, Australia8, Turkey9, and Greece10. The improper and widespread use of phosphine has led to the build-up of resistance levels in several stored insect species5. The increasing levels of resistance warrant increased usage of fumigants which have raised concerns about safety storage of food grains. Additionally, insect resistance contributes to economic damage affecting the grain's market price, nutritional efficiencies and poses threat to global food security11.
Stored grain insect possesses both ‘strong’ and ‘weak’ resistance to phosphine, particularly in T. cataneum and R. dominica8. The detailed genetic study of these two species identified two loci (rph1 and rph 2) conferring the resistance development8,12. The gene for strong resistance was identified as the metabolic enzyme dihydrolipoamide dehydrogenase (DLD) in T. cataneum and R. dominica13. Certain point mutations in DLD are associated with strong resistance to phosphine in insects13.
Being heterothermic organisms, insects have evolved with various adaptations to avoid phosphine toxicity14. As respiratory poison, phosphine toxicity inferred oxidative damage and oxidative stress resulting in aerobic cellular metabolism, which implies producing pro-oxidant substances, viz., reactive oxygen species (ROS)15. ROS alters cells function by damaging the oxidation of nuclear and mitochondrial DNA, lipids, and proteins15. However, insects counteract the deleterious effect of ROS through the induction of antioxidant systems to maintain physiological homeostasis16. The antioxidant enzymes include superoxide dismutase (SOD), catalase (CAT), and peroxidase (POX)17. The SOD helps in the conversion of superoxide anion (O2·−) into oxygen (O2) and hydrogen peroxide (H2O2). Further, CAT and POX dissociate H2O2 into water (H2O) and oxygen (O2)18.
Regularly monitoring phosphine resistance in stored commodities insects is indispensable for ensuring its use’s sustainability and food security in the long run6. Further, the prevalence of phosphine resistance is hasty and staggered in T. castaneum globally. Although resistance to phosphine is granted a significant issue, detailed information about the level of phosphine resistance is scanty. Nonetheless, little is known about the variability of antioxidant defences to counteract the ROS for building tolerance to phosphine toxicity in T. castaneum. Hence, we conducted studies to assess the phosphine susceptibility in field populations of T. castaneum and examined its association with antioxidant enzyme activities, viz., SOD, POX, and CAT using appropriate statistical tools such as Pearson’s Correlation matrix and Principal Component Analysis. The outcome of this would help in increasing our understanding on the oxidative stress associated with phosphine tolerance in insects and may aid in developing sustainable management practices for this cosmopolitan stored pest.
Materials and methods
The present experiment is conducted at the Division of Entomology, ICAR- Indian Agricultural Research Institute, New Delhi (28.6418N, 77.1695E, 246.63 ± 6 m).
Insect populations and culture
Six populations of T. castaneum were collected from different states across India (Table 1; Fig. 1) and one lab population was considered for the susceptible check. The collection areas were grain storage sites and local markets. Insects were collected with their respective hosts. The Tribolium population was identified based on morphological parameters (unlike the confused flour beetle, the antennae of the red flour beetle are distinctly clubbed gradually having three-segmented structure) and as well by using mtCO1 markers. The collected populations were separated from the host in the laboratory and reared in 1.5 L glass bottles on a mixture of 95% all-purpose wheat flour and 5% brewer’s yeast (wt:wt). The rearing chamber was maintained at 30 ± 1 °C and 65 ± 5% relative humidity with a photoperiod of 16:8 (L:D).
Location map of the T. castaneum collection sites. Location map of the study area. Collection sites are indicated in numerical letters and markings (1: Gurgaon, 2: Malwinder Singh, 3: East Kameng, 4: Kailashahar, 5: Mayurbhanj, 6: Mirzapur, 7: Lab susceptible); This map was prepared using the software, ArcGIS version 10.4.
Phosphine generation and bioassay
Phosphine gas (PH3) was generated in a gas burette using the commercial formulation of aluminum phosphide (AL2P3) tablet viz., 56% (F) (Quickphos*) suspended in 5% sulphuric acid solution19. Mortality responses to phosphine were measured against a range of PH3 concentrations viz., 0 to 1.5 mg L−1, including control. Adult beetles (mixed sexes; two weeks old) were used for bioassay. Adults were kept in plastic vials (30 mL; 4.5 cm height × 1.2 cm diameter). The vials were covered with a white muslin cloth, properly labelled, and transferred to air-tight gas desiccators (phosphine chamber).
There were 30 adults per vial representing one replication. Three replications were maintained for each concentration. The desired amount of phosphine was administered by a gas-tight Hamilton syringe through septa in the lid of the desiccator. After an exposure period of 20 h, the insects were removed from the desiccators and transferred to a fresh vial provided with food. A control set was maintained by injecting air into the desiccator instead of phosphine. The fumigation experiments were conducted at 30 ± 1 °C and 65 ± 5% RH. Mortality was recorded after 14 days from the end of the exposure period. The moribund/immobile insects were considered dead for study. The experiment was done in a completely randomized design with three replications for each population. A laboratory population (susceptible check, maintained up to 30 generations) was kept to calculate the resistance ratio.
Assessment of resistance and resistance ratio estimation
Adult beetles (14 days old) from laboratory-reared field populations were used to determine the resistance level. The mortality data obtained through bioassays (as mentioned in the previous section) were subjected to probit analysis to determine lethal concentrations viz., LC50, LC90, and LC9919. The lethal concentrations of phosphine obtained for the lab population were used to calculate the resistance ratio.
Enzyme assays
Tissue processing
The antioxidant enzyme activities viz., Superoxide Dismutase (SOD), Peroxidase (POX), and Catalase were measured in the field populations of T. castaneum20. The adult beetles of the test populations were exposed to LC50 concentration for 20 h and surviving beetles were used for enzyme assays. The survived beetles were homogenized with 100 µL of 50 mM phosphate buffer (pH 7.0) in microcentrifuge tubes (1.5 mL). Homogenization was done using a motorized pellet pestle (Fisher Scientific) in a mini-cooler. The homogenates were centrifuged at 16,000 rpm for 10 min at 4 °C (Eppendorf, Centrifuge 5425R, Germany). The supernatants were used as enzyme extracts for assays.
Enzymatic activities
Catalase activity was measured using the method described by Beer and Sizer21 with minor modifications. The activity was measured as a decrease in absorbance at 240 nm for 3 min using H2O2 (20 mM, final concentration) as the substrate (ε = 39.4 L M−1 cm−1). Peroxidase activity was determined as described by Armstrong et al.22 with minor modifications. 0.1 M concentration of H2O2 was taken as substrate. Absorbance was recorded at 470 nm for 5 min (ε = 26.6 L M−1 cm−1). CAT and POX activities were expressed as μmol H2O2 reduced per minute per milligram of protein (μmol min−1 mg−1). A solution without enzyme extract was considered blank. SOD activity was assessed by the method described by Kakkar23. Briefly, the assay mixture contained sodium pyrophosphate buffer (0.052 M), phenazine methosulphate, nitroblue tetrazolium, reduced nicotinamide adenine dinucleotide, and enzyme lysate. The principle relies on the inhibition of the formation of NADH-phenazine methosulfate-nitroblue tetrazolium formazan. Enzyme activity was measured at 560 nm and expressed in terms of the rate of percentage inhibition. One unit of SOD is defined as the amount of enzyme needed to exhibit 50% dismutation of the superoxide radical. All enzymatic activities were estimated with a UV–VIS EPOCH 2 microplate reader spectrophotometer at 25 °C (BioTek, USA) using microplates (Tarsons).
Protein estimation
Total protein concentration was determined using the Bradford method (Bradford 1976)24 using bovine serum albumin as standard, and the absorbance was recorded at 595 nm.
Statistical analysis
The corrected mortality data were subjected to probit analysis (Polo Plus, Ver. 2.0 Leora Software 2002) to determine LC50, LC90, and LC99 values and fiducial limits. The Chi-square test was performed to ascertain the goodness of fit25. The insect collection sites are depicted in a map using ArcGIS 10.4 software. Analysis of variance and Duncan’s Multiple Range Tests (DMRT) were conducted using the statistical package of “R” of version 4.1.2. Values are presented in the table as the means of the different locations with standard error of means (SEM). Pearson’s Correlations among the traits were displayed using the “Metan” package. PCA biplots analysis used “FactoMineR” and “Factoextra” packages in R for dimension reduction.
Results
Toxicity of phosphine to different populations of T. castaneum
The collection sites of all T. castaneum populations used in this study are shown in Fig. 1. The bioassay study was conducted to generate dose–response data for the T. castaneum against phosphine. The results of the dose–response regressions analyzed by probit assay were shown in Table 2. The mortality data obtained for different T. castaneum populations when exposed to a range of PH3 concentrations for 20 h at 30 °C, revealed substantial variation in susceptibility to phosphine in these populations as compared to the susceptible lab population. The calculated χ2 value was lesser than the table value (χ2 = 14.067, df = 7) at a 5% significance level, symbolizing population homogeneity (Table 2). Henceforth, the probit model was considered to be appropriate. The tested T. castaneum populations exhibited varying levels of slopes for probit response curves (0.961 to 1.645) for phosphine. The susceptibility of the populations (LC50) ranged from 0.038 to 1.277 mg L−1 (Table 2). The Mirzapur population was the least susceptible to phosphine fumigation, with an LC50 value of 1.227 mg L−1, compared to the lab population with an LC50 value of 0.018 mg L−1. Two T. castaneum populations from Kailashahar and East Kameng were highly susceptible to phosphine with the LC50 values being 0.038 mg L−1 and 0.043, respectively. Comparatively, populations from Malwinder Singh and Gurgaon were found to be lower susceptibility with LC50 values of 0.825 and 0.881 mg L−1, respectively. Similarly, the LC90 and LC99 values ranged from 0.075 to 27.546 mg L−1 and 0.239 to 336.96 mg L−1 across the population (Table 2). Moreover, the dose–response curve depicts the percentage of mortality across the population to phosphine exposure (Fig. 2). There was upto thirty-four-fold increase in resistance ratio in Mirzapur with comparison to the most susceptible population viz., Kailashahar-2.11%. However, the magnitude of resistance was recorded as 70.94-fold in comparison to the lab population.
Probit analysis of T. castaneum to dose–response mortality. The mortality response of different populations after exposure to phosphine. The dose–response lines of each population were drawn using a probit linear model y = αx + β in which α and β are the slope and intercept, respectively; R2 value is depicted. x is the log-transformed dose (mg L−1), y is the percent mortality; Locations; (a) Gurgaon, (b) Malwinder Singh, (c) East Kameng, (d) Kailashahar, (e) Mayurbhanj, (f) Mirzapur, (g) Lab susceptible.
The specific activity of antioxidant enzymes in different T. castaneum populations
Superoxide dismutase activity
The activity of SOD was induced following phosphine exposure. The activity ranged from 8.77 to 18.82 U mg−1 protein, with a significant level of variation (F value = 72.66, df = 6, p < 0.001) across the population (Table 3). The study revealed that the Mirzapur population showed the highest SOD activity (18.82 U mg−1 protein) compared to the laboratory-susceptible population (5.00 U mg−1 protein). Gurgaon and Malwinder Singh populations exhibited relatively lower activity, 17.16 and 15.99 U mg−1 protein respectively. The SOD parameter was found to be 12.84, 10.66, and 8.77 U mg−1 protein in Kailashahar, Mayurbhanj, and East Kameng compared to the reference lab population, respectively.
Peroxidase activity
The specific activity of Peroxidase differed significantly among the populations of T. castaneum (F value = 180.294, df = 6, p < 0.001), with strength ranging from 82.19 to 408.320 µM H2O2 reduced min−1 mg−1 of protein (Table 3). The East Kameng and Kailashahar populations had shown lesser peroxidase activity, i.e., 82.192 and 86.737 µM min−1 mg−1, respectively. The highest peroxidase activity was observed in the Mirzapur population (408.320 µM min−1 mg−1) in comparison to the lab-susceptible population (52.42 µM min−1 mg−1).
Catalase activity
A significant difference prevailed in catalase activity across collected T. castaneum populations (F value = 26.566, df = 6, p < 0.001), (Table 3). The activity ranged from 61.11 to 231.28 µM H2O2 reduced min−1 mg−1 of protein. Catalase activity escalated in the northeast populations of Kailashahar (231.28 µM min−1 mg−1) and East Kameng (217.77 µM min−1 mg−1) in comparison to lab susceptible check (247.49 µM min−1 mg−1). On the contrary, we observed the lowest activity value in the Mirzapur population (61.11 µM min−1 mg−1).
Correlation of phosphine toxicity with antioxidant enzyme activities
The strength of the antioxidant enzymes and resistance level (RR) of different T. castaneum populations was analyzed through a Pearson’s correlation model (Fig. 3a). Our study highlighted the significant influence of phosphine exposure on CAT, POX, and SOD activities in all T. castaneum populations examined in this study. The values of Pearson’s correlation coefficients were positively linked with the resistant ratio factor of different T. castaneum populations for POX (r = 0.98, p < 0.001) and SOD (r = 0.89, p < 0.05) and observed significant variability. Conversely, the CAT activity was negatively associated with the RR factor with r = − 0.98 at p < 0.001 (Fig. 3a). The regression analysis used the resistant factor as an independent variable and the antioxidant enzymes as the dependent variable. The R2 values for POX, SOD, and CAT are recorded as 0.96, 0.79, and 0.96, respectively (Fig. 3b–d). Furthermore, the slopes are positively associated with SOD and POX, whereas CAT negatively negates with resistance ratio (Fig. 3). Our study found the susceptibility of populations increased with decreased SOD and POX activity.
Correlation and regression analysis of resistance ratio (RR) with the antioxidant enzymes: SOD, POX, and CAT in T. castaneum. (a) Correlation of RR with SOD, POX, and CAT; (b–d) regression equation of RR with CAT, POX, and SOD, respectively. The shaded area in the regression analysis represents the 95% confidence interval. The R2 value is also mentioned. Signif. codes: 0 ‘***’ 0.001 ‘**’ 0.01 ‘*’ 0.05 ‘.’ 0.1 ‘’ 1.
PCA based on toxicity data and the specific activity of antioxidant enzymes indicated that the first principal component contributed 95% variation (Fig. 4a); the significant contributors are POX, CAT, and LC50. (Fig. 4b). The second dimension represents the 4.1% variation receiving contribution only from the SOD parameter (Fig. 4c). Our study revealed that the eigenvalue was 3.80 and 0.16 for dimensions one and two, respectively (Table 4). The traits POX, SOD, and LC50 were distributed with an acute angle, indicating a positive relationship, whereas the CAT showed a negative association with all the studied traits (Fig. 4). All the components having similar lengths symbolized a good amount of information to component contribution (Fig. 4).
PCA biplot and contribution of studied traits to dimensions in T. castaneum. (a) PCA biplot, Presentation of four variables viz., LC50, CAT, POX, and SOD; (b,c) contribution of LC50, CAT, POX, and SOD to dimensions one and two, respectively; PC1 and PC2 are represented on the horizontal and vertical axes, with a cumulative variance of 95% and 4.1%, respectively.
The grouping of the population is depicted in Fig. 5. The least susceptible (Mirzapur) and most susceptible (Kailashahar) were opposite to each other are distinctly presented (Fig. 5). Malwinder Singh and Gurgaon populations showed lower susceptibility and were grouped in the biplot, corresponding with POX and SOD. Moreover, the biplot depicts the higher susceptible populations to phosphine, viz., East kameng and Mayurbhanj having lower LC50 was close to each other (Fig. 5). In addition, those populations were more closed to catalase depicting higher catalase activity (Fig. 3a).
PCA analysis considering locations and all traits (LC50, CAT, POX, and SOD). Visualization of correlation showing the influence of antioxidant enzymes on the LC50 of the populations used in this study; Black dots (●) in the figure represent active observations referring to different populations in the study. The distance of the variables from the center indicates the magnitude of influence; PC1 and PC2 are represented on the horizontal and vertical axes, with a cumulative variance of 95% and 4.1%, respectively.
Discussion
Phosphine as a fumigant is extensively used to manage the stored grain pest worldwide, as it is cost-effective and leaves no harmful residue on commodities26. The present study gives the current phosphine susceptibility/resistance levels in contemporary field populations of T. castaneum collected across India. A differential susceptibility to phosphine as reported in this study (Table 2) had earlier been documented in key grain pests globally8. The occurrence of varying levels of susceptibility to phosphine has earlier been reported in T. castaneum from India27, in Trogoderma granarium from Pakistan28,29 and in Sitophilus zeamais from Brazil30. Compared to an earlier report31, there has been a significant loss of susceptibility to phosphine implying the widespread prevalence of phosphine resistance in the contemporary field populations of T. castaneum.
The over-dependency upon phosphine and inappropriate fumigation practices might have triggered the build-up resistance to phosphine in key stored product insect pests across Asia, Africa, and South America32,33,34. Henceforth, this study revealed the burgeoning issue of phosphine administration in diverse storage conditions. The prevalence of phosphine resistance might be due to resistance genes in the population or by the selection pressure to phosphine stress35. The variability in the resistance ratio among the different populations within the same species, as observed in the study, might be due to their genetic diversity13,36 and distinct geographical territories37.
Our probit analysis found LC50 at 0.038 and 1.277 mg L−1 for the high and least susceptible populations, including Kailashahr and Mirzapur (Table 2). Compared to an earlier report, there have been consistent findings were observed regarding phosphine resistance (having lowest median dose was at 0.358 and 1.901 mg L−1 in the Gohana and Ajmeer) in the contemporary populations of T. castaneum in India31. However, an elevated range of LC50 was reported in T. castaneum populations from Pakistan38. In Oklahoma, USA, the study found that the LC99 value is 9.2 and 6.6 ppm for resistant T. castaneum and R. dominica populations, respectively6. However, after two decades, Opit et al.7 described that higher level of resistance in both the populations of Oklahoma, viz. T. casataneum (377.49 ppm) and R. dominica (3430.8 ppm). This highlights the escalation of resistance/diminishing susceptibility in the same population within a territory during a specific period, though our study outcomes are inconsistent with those findings.
The molecular analysis identified that the metabolic enzyme dihydrolipoamide dehydrogenase (DLD) was tightly linked to a phosphine resistance gene i.e., rph2 in T. castaneum and other stored product insect pests13. However, recent investigations have highlighted the role of oxidative stress associated with antioxidant enzymes in phosphine toxicity39.
PH3, being a respiratory poison, induces oxidative damage by producing reactive oxygen species (oxygen-derived radicals), strongly contributing to phosphine toxicity40. Phosphine-induced oxidative stress was reported in many arthropods41,42, and mammals43,44. However, insects have a comprehensive antioxidant defence system to relieve oxidative stress caused by phosphine toxicity. Our results demonstrated increased activity of SOD and peroxidase with decreased susceptibility (Table 3). On contrary, the catalase activity was enhanced with increasing susceptibility (Table 3). SOD eliminates the ROS by the dismutation of superoxide anion to hydrogen peroxide through the catalytic process45. Our study found SOD activity was higher in Mirzapur (18.82 U mg−1 protein) followed by Gurgaon (17.16 U mg−1 protein) and Malwinder Singh (15.99 U mg−1 protein) population. Inversely, lower activity of SOD was found in higher susceptibility populations viz., East Kameng, Mayurbhanj, and kailashahar that are exhibiting lower LC50 values (Table 3). Liu et al.46 demonstrated that the decreased activity of SOD in phosphine-susceptible insect populations supports our findings. However, phosphine susceptibility in stored insects is associated with enhanced oxidative metabolism under low oxygen (hypoxic) conditions47. On the contrary, the resistant population absorbs lesser phosphine to reduce oxygen metabolism30.
Catalase is an essential enzyme that detoxifies hydrogen peroxide to water. The catalase activity was positively linked with phosphine resistance in T. castaneum48. On the contrary, our findings revealed high catalase activity in susceptible populations such as the highly susceptible Kailashahar population (Table 3). We speculate that the reduced catalase activity in the least susceptible population of T. castaneum was probably attributed to decreased toxicant absorption. Bolter and Chefurka49 had earlier observed that the granary weevil, Sitophilus granarius population showing susceptibility to phosphine was having high catalase activity. The results of our study conform with this finding. In addition, a transcriptional study in Drosophila melanogaster revealed that the catalase gene was inhibited under phosphine stress conditions46. It curtails that phosphine is not directly inactivating its target enzymes but suppresses them by interfering with a complex signal transduction process46.
Though the peroxidase functions similarly to catalase, our findings revealed reduced activity of this enzyme in phosphine-susceptible populations (Table 3). Our results are consistent with that of Ranjith et al.20. On the contrary, Bolter and Chefurka49 observed reduced peroxidase activity in the phosphine-resistant S. granarius population. Similarly, the increased POX activity was noticed among R. dominica populations showing increased susceptibility to phosphine41. Yadav et al.50 observations on the activity of SOD and POX in T. granarium in response to phosphine also support our findings.
A significant positive correlation of SOD (r = 0.89) and POX (r = 0.98) with resistance ratio was observed in our study (Fig. 3a). Our results corroborated with an earlier report42. PCA biplot analysis (Fig. 4a) deduced the strong positive association of SOD and POX activities with the T. castaneum populations showing resistance to phosphine while catalase activity was negatively associated (Fig. 4b). The reduced activity of POX in susceptible populations could be due to the overproduction of H2O2 leading to cell oxidative damage. The increased activity of SOD and POX in resistant populations might have helped mitigate the negative impact of oxyradicals. Our result corroborated well with the earlier investigations by Yadav et al.50 and Ranjith et al.20 on T. granarium and R. dominica, respectively.
However, the cause of varied responses of antioxidant enzymes to phosphine toxicity in susceptible and resistant populations needs to be explored. Genomic and molecular investigations may elucidate in detail the cellular antioxidant mechanism in insects under xenobiotic stress.
Conclusion
In conclusion, this study provided insight into the prevalence of phosphine resistance in the contemporary T. castaneum populations across India and associated antioxidant enzyme modulation. The study's indication of oxidative stress as a contributor to resistance presents an opportunity for heightened examination. An in-depth inquiry into the mechanistic intricacies of how these enzymes function in response to phosphine-induced stress, coupled with their putative involvement in detoxification pathways can substantially enrich the study's scientific rigor and conceptual coherence.
Data availability
All data have been provided in the manuscript.
References
Rajashekar, Y., Gunasekaran, N. & Shivanandappa, T. Insecticidal activity of the root extract of Decalepis hamiltonii against stored product insect pests and its application in grain protection. J. Food Sci. Technol. 47(3), 310–314 (2010).
Hagstrum, D. W. & Phillips, T. W. Evolution of stored-product entomology: protecting the world food supply. Annu. Rev. Entomol. 62, 379–397 (2017).
El-Mofty, M. M., Sakr, S. A., Osman, S. I. & Toulan, B. A. Carcinogenic effect of biscuits made of flour infested with Tribolium castaneum in Bufo regularis. Oncology 46(1), 63–65 (1989).
Bell, C. H. Fumigation in the 21st century. Crop. Prot. 19, 563–569 (2000).
Nayak, M. K., Daglish, G. J., Phillips, T. W. & Ebert, P. R. Resistance to the fumigant phosphine and its management in insect pests of stored products: A global perspective. Annu. Rev. Entom. 65, 333–350 (2020).
Zettler, J. L. & Cuperus, G. W. Pesticide resistance in Tribolium castaneum (Coleoptera: Tenebrionidae) and Rhyzopertha dominica (Coleoptera: Bostrichidae) in wheat. J. Econ. Entomol. 83, 1677–1681 (1990).
Opit, G. P., Phillips, T. W., Aikins, M. J. & Hasan, M. M. Phosphine resistance in Tribolium castaneum and Rhyzopertha dominica from stored wheat in Oklahoma. J. Econ. Entomol. 105, 1107–1114 (2012).
Jagadeesan, R., Collins, P. J., Daglish, G. J., Ebert, P. E. & Schlipalius, D. I. Phosphine resistance in the rust red flour beetle, Tribolium castaneum (Coleoptera: Tenebrionidae): inheritance, gene interactions and fitness costs. PLoS ONE 7, 1–12 (2012).
Koçak, E., Yilmaz, A., Alpkent, Y. N. & Ertürk, S. Phosphine resistance to some coleopteran pests in stored grains across Turkey. In Proc Of the 11th Int. Conf. on the IOBC-WPRS Bulletin (eds. Trematerra, P. & Trdan, S.) Vol. 130 303–310 (2018).
Agrafioti, P., Athanassiou, C. G. & Nayak, M. K. Detection of phosphine resistance in major stored-product insects in Greece and evaluation of a field resistance test kit. J. Stored Prod. Res. 82, 40–47 (2019).
Thakore, Y. The biopesticide market for global agricultural use. Ind. Biotechnol. 2(3), 194–208 (2006).
Mau, Y. S., Collins, P. J., Daglish, G. J., Nayak, M. K. & Ebert, P. R. The rph2 gene is responsible for high level resistance to phosphine in independant field strains of Rhyzopertha dominica. PLoS ONE 7, e34027 (2012).
Schlipalius, D. I. et al. A core metabolic enzyme mediates resistance to phosphine gas. Science 338, 807–810 (2012).
Paul, A., Radhakrishnan, M., Anandakumar, S., Shanmugasundaram, S. & Anandharamakrishnan, C. Disinfestation techniques for major cereals: A status report. Compr. Rev. Food Sci. Food Saf. 19(3), 1125–1155 (2020).
Faggio, C., Pagano, M., Alampi, R., Vazzana, I. & Felice, M. R. Cytotoxicity, haemolymphatic parameters, and oxidative stress following exposure to sub-lethal concentrations of quaternium-15 in Mytilus galloprovincialis. Aquat. Toxicol. 180, 258–265. https://doi.org/10.1016/j.aquatox.2016.10.010 (2016).
Pena-Ahumada, A., Kahmann, U., Dietz, K. J. & Baier, M. Regulation of peroxiredoxin expression versus expression of Halliwell-Asada-Cycle enzymes during early seedling development of Arabidopsis thaliana. Photosynth. Res. 89(2), 99–112. https://doi.org/10.1007/s11120-006-9087-3 (2006).
Ighodaro, O. & Akinloye, O. First line defence antioxidants-superoxide dismutase (SOD), catalase (CAT) and glutathione peroxidase (GPX): their fundamental role in the entire antioxidant defence grid. Alexandria J. Med. 54(4), 287–293 (2018).
Bhattacharjee, S. Reactive Oxygen Species in Plant Biology 107–125 (Springer India, 2019).
FAO. Recommended methods for the detection and measurement of resistance of agricultural pests to pesticides: Tentative method for adults of some stored cereals, with methyl bromide and phosphine: Method FAO n.16. FAO Plant Prot. Bull. 23, 12–25 (1975).
Ranjith, H. V., Sagar, D., Kalia, V. K., Dahuja, A. & Subramanian, S. Differential activities of antioxidant enzymes, superoxide dismutase, peroxidase, and catalase vis-à-vis phosphine resistance in field populations of lesser grain borer (Rhyzopertha dominica) from India. Antioxidants 12, 270. https://doi.org/10.3390/antiox12020270 (2023).
Beer, R. F. & Sizer, I. W. A. spectrophotometric method for measuring the breakdown of hydrogen peroxide by catalase. J. Biol. Chem. 195, 133–140 (1952).
Armstrong, D., Rinehart, R., Dixon, L. & Reigh, D. Changes of peroxidase with age in Drosophila. J. Age 1, 8–12 (1978).
Kakkar, P., Das, B. & Vishwanathan, P. N. A modified spectrophotometric assay of superoxide dismutase. Indian J. Biochem. Biophys. 21, 130–132 (1984).
Bradford, M. M. A rapid and sensitive method for the quantitation of microgram quantities of protein utilizing the principle of protein-dye binding. Anal. Biochem. 72, 248–254 (1976).
Finney, D. J. Probit Analysis 3rd edn, 333 (Cambridge University Press, 1971).
Sciuto, A. M., Wong, B. J., Martens, M. E., Hoard-Fruchey, H. & Perkins, M. W. Phosphine toxicity: A story of disrupted mitochondrial metabolism. Ann. N. Y. Acad. Sci. 1374, 41–51 (2016).
Rajendran, S. Inhibition of hatching of Tribolium castaneum by phosphine. J. Stored Prod. Res. 36, 101–106 (2000).
Ahmedani, M. S., Shaheen, N., Ahmedani, M. Y. & Aslam, M. Status of phosphine resistance in khapra beetle, Trogoderma granarium (Everts) strains collected from remote villages of Rawalpindi district. Pak. Entomol. 29, 95–102 (2007).
Riaz, T., Shakoori, F. R. & Ali, S. S. Toxicity of phosphine against tolerant and susceptible populations of Trogoderma granarium collected from Punjab, Pakistan. Punjab Univ. J. Zool. 31, 2530 (2016).
Pimentel, M. A. G., Faroni, L. R. D. A., Guedes, R. N. C., Sousa, A. H. & Tótola, M. R. Phosphine resistance in Brazilian populations of Sitophilus zeamais Motschulsky (Coleoptera: Curculionidae). J. Stored Prod. Res. 45, 71–74 (2009).
Ramya, R. S., Srivastava, C. & Subramanian, S. Monitoring of phosphine resistance in Indian populations of Tribolium castaneum (Herbst) from stored wheat. Indian J. Entomol. 80, 19–23 (2018).
Lorini, I., Collins, P. J., Daglish, G. J., Nayak, M. K. & Pavic, H. Detection and characterisation of strong resistance to phosphine in Brazilian Rhyzopertha dominica (F.) (Coleoptera: Bostrychidae). Pest Manag. Sci. 63, 358–364 (2007).
Pimentel, M. A. G., Farroni, L. R. D. A., da Silva, F. H., Batista, M. D. & Guedes, R. N. C. Spread of phosphine resistance among Brazilian populations of three species of stored product insects. Neotrop. Entomol. 39, 101–107 (2010).
Emery, R. N., Nayak, M. K. & Holloway, J. C. Lessons learned from phosphine resistance monitoring in Australia. Stewart Postharvest. Rev. 3, 6 (2011).
Hernandez Nopsa, J. F. et al. Ecological networks in stored grain: Key postharvest nodes for emerging pests, pathogens, and mycotoxins. BioScience 65, 985–1002 (2015).
Nguyen, T. T., Collins, P. J., Duong, T. M., Schlipalius, D. I. & Ebert, P. R. Genetic conservation of phosphine resistance in the rice weevil Sitophilus oryzae (L.). J. Hered. 107, 228–237 (2016).
Cato, A. J., Elliott, B., Nayak, M. K. & Phillips, T. W. Geographic variation in phosphine resistance among North American populations of the red flour beetle. J. Econ. Entomol. 110, 1359–1365 (2017).
Wakil, W., Kavallieratos, N. G., Usman, M., Gulzar, S. & El-Shafie, H. A. Detection of phosphine resistance in field populations of four key stored-grain insect pests in Pakistan. Insects 12(4), 288 (2021).
Nath, N. S., Bhattacharya, I., Tuck, A. G., Schlipalius, D. I. & Ebert, P. R. Mechanism of phosphine toxicity. J. Toxic. https://doi.org/10.1155/2011/494168 (2011).
Sohn, H. Y., Kwon, C. S., Kwon, G. S., Lee, J. B. & Kim, E. Induction of oxidative stress by endosulphan and protective effect of lipid-soluble anti-oxidants against endosulphan-induced oxidative damage. Toxicol. Lett. 151, 357–365 (2004).
Chaudhry, M. Q. & Price, N. R. Comparison of the oxidant damage induced by phosphine and the uptake and tracheal exchange of 32P-radiolabelled phosphine in the susceptible and resistant strains of Rhyzopertha dominica (F.) (Coleoptera: Bostrychidae). Pestic. Biochem. Physiol. 42, 167–179 (1992).
Valmas, N., Zuryn, S. & Ebert, P. R. Mitochondrial uncouplers act synergistically with the fumigant phosphine to disrupt mitochondrial membrane potential and cause cell death. Toxicology 252, 33–39 (2008).
Hsu, C. H., Han, B., Liu, M., Yeh, C. & Casida, J. E. Phosphine-induced oxidative damage in rats: attenuation by melatonin. Free Radic. Biol. Med. 28, 636–642 (2000).
Hsu, C. H., Quistad, G. B. & Casida, J. E. Phosphine induced oxidative stress in Hepa 1c1c7 cells. Toxicol. Sci. 46, 204–210 (1998).
Finkel, T. & Holbrook, N. J. Oxidants oxidative stress and the biology of ageing. Nature 408, 239–247 (2000).
Liu, T., Li, L., Zhang, F. & Wang, Y. Transcriptional inhibition of the Catalase gene in phosphine-induced oxidative stress in Drosophila melanogaster. Pestic. Biochem. Phys. 124, 1–7 (2015).
Price, N. R. & Dance, S. J. Some biochemical aspects of phosphine action and resistance in three species of storage product beetles. Comp. Biochem. Physiol. 16, 277–281 (1983).
Gao, X. W. Comparison of specific activity of catalase in the phosphine-resistant and phosphine-susceptible strains of red flour beetle Tribolium castaneum (Herbst) (2009).
Bolter, C. J. & Chefurka, W. The effect of phosphine treatment on superoxide dismutase, catalase, and peroxidase in the granary weevil, Sitophilus granarius. Pestic. Biochem. Physiol. 36, 52–60 (1990).
Yadav, S. K., Srivastava, C. & Sabtharishi, S. Phosphine resistance and antioxidant enzyme activity in Trogoderma granarium Everts. J. Stored Prod. Res. 87, 101636 (2020).
Acknowledgements
The authors gratefully acknowledge the Division of Entomology, Indian Council of Agricultural Research-Indian Agricultural Research Institute, New Delhi, for providing the necessary facilities. The first author is supported by the Central Horticultural Experiment Station (ICAR-Indian Institute of Horticultural Research), Bhubaneswar. The guidance of the advisory committee is highly acknowledged. We are also grateful for the help rendered by field staff while collection of the insect samples at study locations.
Funding
Funding support in the form of IARI Student Fellowship to the first author and Research Grant to the corresponding author from the Department of Biotechnology, Government of India and IARI, NAHEP- CAAST programme is gratefully acknowledged.
Author information
Authors and Affiliations
Contributions
S.S.: Methodology, Data curation, Formal analysis, Investigation, Software, Writing—original draft, editing; S.M.N.: Methodology, Analysis, review & editing; S.T.: Software, Statistical analysis; D.S.: review & editing. S.S.N.: Methodology, Investigation, Resources, Conceptualization, Writing—review & editing, Visualization Supervision, Project administration, Funding acquisition.
Corresponding author
Ethics declarations
Competing interests
The authors declare no competing interests.
Additional information
Publisher's note
Springer Nature remains neutral with regard to jurisdictional claims in published maps and institutional affiliations.
Rights and permissions
Open Access This article is licensed under a Creative Commons Attribution 4.0 International License, which permits use, sharing, adaptation, distribution and reproduction in any medium or format, as long as you give appropriate credit to the original author(s) and the source, provide a link to the Creative Commons licence, and indicate if changes were made. The images or other third party material in this article are included in the article's Creative Commons licence, unless indicated otherwise in a credit line to the material. If material is not included in the article's Creative Commons licence and your intended use is not permitted by statutory regulation or exceeds the permitted use, you will need to obtain permission directly from the copyright holder. To view a copy of this licence, visit http://creativecommons.org/licenses/by/4.0/.
About this article
Cite this article
Singh, S., Nebapure, S.M., Taria, S. et al. Current status of phosphine resistance in Indian field populations of Tribolium castaneum and its influence on antioxidant enzyme activities. Sci Rep 13, 16497 (2023). https://doi.org/10.1038/s41598-023-43681-y
Received:
Accepted:
Published:
DOI: https://doi.org/10.1038/s41598-023-43681-y
Comments
By submitting a comment you agree to abide by our Terms and Community Guidelines. If you find something abusive or that does not comply with our terms or guidelines please flag it as inappropriate.