Abstract
Sub-cortical volumetric differences were associated with attention-deficit/hyperactivity disorder (ADHD) in a recent multi-site, mega-analysis of 1713 ADHD persons and 1529 controls. As there was a wide range of effect sizes among the sub-cortical volumes, it is possible that selective neuronal vulnerability has a role in these volumetric losses. To address this possibility, we used data from Allen Brain Atlas to investigate variability in gene expression profiles between subcortical regions of typically developing brains. We tested the hypothesis that the expression of genes in a set of curated ADHD candidate genes and five a priori selected, biological pathways would be associated with the Enhancing NeuroImaging Genetics through Meta-Analysis (ENIGMA) findings. Across the subcortical regions studied by ENIGMA, gene expression profiles for three pathways were significantly correlated with ADHD-associated volumetric reductions: apoptosis, oxidative stress and autophagy. These correlations were strong and significant for children with ADHD, but not for adults. Although preliminary, these data suggest that variability of structural brain anomalies in ADHD can be explained, in part, by the differential vulnerability of these regions to mechanisms mediating apoptosis, oxidative stress and autophagy.
This is a preview of subscription content, access via your institution
Access options
Subscribe to this journal
Receive 12 print issues and online access
$259.00 per year
only $21.58 per issue
Buy this article
- Purchase on Springer Link
- Instant access to full article PDF
Prices may be subject to local taxes which are calculated during checkout


Similar content being viewed by others
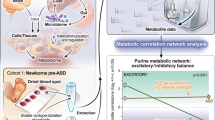
References
Faraone SV, Asherson P, Banaschewski T, Biederman J, Buitelaar JK, Ramos-Quiroga JA et al. Attention-deficit/hyperactivity disorder. Nat Rev Dis Primers 2015; 1: 15020.
Banerjee TD, Middleton F, Faraone SV. Environmental risk factors for attention-deficit hyperactivity disorder. Acta Pediatr 2007; 96: 1269–1274.
Rubia K, Alegria AA, Brinson H. Brain abnormalities in attention-deficit hyperactivity disorder: a review. Rev Neurol 2014; 58(Suppl 1): S3–S16.
Valera EM, Faraone SV, Murray KE, Seidman LJ. Meta-analysis of structural imaging findings in attention-deficit/hyperactivity disorder. Biol Psychiatry 2007; 61: 1361–1369.
Spencer TJ, Brown A, Seidman LJ, Valera EM, Makris N, Lomedico A et al. Effect of psychostimulants on brain structure and function in ADHD: a qualitative literature review of magnetic resonance imaging-based neuroimaging studies. J Clin Psychiatry 2013; 74: 902–917.
Hoogman M, Bralten J, Hibar DP, Mennes M, Zwiers MP, Schweren LS et al. Subcortical brain volume differences in participants with attention deficit hyperactivity disorder in children and adults: a cross-sectional mega-analysis. Lancet Psychiatry 2017; 4: 310–319.
Wang X, Michaelis EK. Selective neuronal vulnerability to oxidative stress in the brain. Front Aging Neurosci 2010; 2: 12.
Saxena S, Caroni P. Selective neuronal vulnerability in neurodegenerative diseases: from stressor thresholds to degeneration. Neuron 2011; 71: 35–48.
Roselli F, Caroni P. From intrinsic firing properties to selective neuronal vulnerability in neurodegenerative diseases. Neuron 2015; 85: 901–910.
Joseph N, Zhang-James Y, Perl A, Faraone SV. Oxidative stress and attention deficit hyperactivity disorder: a meta-analysis. J Atten Disord 2015; 19: 915–924.
Reus GZ, Scaini G, Jeremias GC, Furlanetto CB, Morais MO, Mello-Santos LM et al. Brain apoptosis signaling pathways are regulated by methylphenidate treatment in young and adult rats. Brain Res 2014; 1583: 269–276.
Thapar A, Martin J, Mick E, Arias Vasquez A, Langley K, Scherer SW et al. Psychiatric gene discoveries shape evidence on ADHD's biology. Mol Psychiatry 2016; 21: 1202–1207.
Poelmans G, Pauls DL, Buitelaar JK, Franke B. Integrated genome-wide association study findings: identification of a neurodevelopmental network for attention deficit hyperactivity disorder. Am J Psychiatry 2011; 168: 365–377.
Neale BM, Medland SE, Ripke S, Asherson P, Franke B, Lesch KP et al. Meta-analysis of genome-wide association studies of attention-deficit/hyperactivity disorder. J Am Acad Child Adolesc Psychiatry 2010; 49: 884–897.
Brookes K, Xu X, Chen W, Zhou K, Neale B, Lowe N et al. The analysis of 51 genes in DSM-IV combined type attention deficit hyperactivity disorder: association signals in DRD4, DAT1 and 16 other genes. Mol Psychiatry 2006; 11: 934–935.
Sunkin SM, Ng L, Lau C, Dolbeare T, Gilbert TL, Thompson CL et al. Allen Brain Atlas: an integrated spatio-temporal portal for exploring the central nervous system. Nucleic Acids Res 2013; 41(Database issue): D996–D1008.
Demmontis D, Walters RK, Matrin J, Mattheisen M, Als TD, Agerbro E et al. Discovery of the first genome-wide significant risk loci for ADHD. BiorXiv 2017.
de Leeuw CA, Mooij JM, Heskes T, Posthuma D. MAGMA: generalized gene-set analysis of GWAS data. PLoS Comput Biol 2015; 11: e1004219.
Zheng J, Erzurumluoglu AM, Elsworth BL, Kemp JP, Howe L, Haycock PC et al. LD Hub: a centralized database and web interface to perform LD score regression that maximizes the potential of summary level GWAS data for SNP heritability and genetic correlation analysis. Bioinformatics 2017; 33: 272–279.
Chen YC, Sudre G, Sharp W, Donovan F, Chandrasekharappa SC, Hansen N et al. Neuroanatomic, epigenetic and genetic differences in monozygotic twins discordant for attention deficit hyperactivity disorder. Mol Psychiatry 2017.
Lushchak VI. Free radicals, reactive oxygen species, oxidative stresses and their classifications. Ukr Biochem J 2015; 87: 11–18.
Turrens JF. Mitochondrial formation of reactive oxygen species. J Physiol 2003; 552(Pt 2): 335–344.
Halliwell B. Reactive oxygen species in living systems: source, biochemistry, and role in human disease. Am J Med 1991; 91: 14S–22S.
Lee J, Giordano S, Zhang J. Autophagy mitochondria and oxidative stress: cross-talk and redox signalling. Biochem J 2012; 441: 523–540.
Bloch MH, Qawasmi A. Omega-3 fatty acid supplementation for the treatment of children with attention-deficit/hyperactivity disorder symptomatology: systematic review and meta-analysis. J Am Acad Child Adolesc Psychiatry 2011; 50: 991–1000.
Chovanova Z, Muchova J, Sivonova M, Dvorakova M, Zitnanova I, Waczulikova I et al. Effect of polyphenolic extract, Pycnogenol, on the level of 8-oxoguanine in children suffering from attention deficit/hyperactivity disorder. Free Radic Res 2006; 40: 1003–1010.
Garcia RJ, Francis L, Dawood M, Lai ZW, Faraone SV, Perl A. Attention deficit and hyperactivity disorder scores are elevated and respond to NAC treatment in patients with SLE. Arthritis Rheum 2013; 65: 1313–1318.
Leffa DT, Bellaver B, de Oliveira C, de Macedo IC, de Freitas JS, Grevet EH et al. Increased oxidative parameters and decreased cytokine levels in an animal model of attention-deficit/hyperactivity disorder. Neurochem Res 2017; 42: 3084–3092.
Fulda S, Gorman AM, Hori O, Samali A. Cellular stress responses: cell survival and cell death. Int J Cell Biol 2010; 2010: 214074.
Nikoletopoulou V, Markaki M, Palikaras K, Tavernarakis N. Crosstalk between apoptosis, necrosis and autophagy. Biochim Biophys Acta 2013; 1833: 3448–3459.
Maday S. Mechanisms of neuronal homeostasis: autophagy in the axon. Brain Res 2016; 1649(Pt B): 143–150.
Lee JA. Neuronal autophagy: a housekeeper or a fighter in neuronal cell survival? Exp Neurobiol 2012; 21: 1–8.
Lv X, Jiang H, Li B, Liang Q, Wang S, Zhao Q et al. The crucial role of Atg5 in cortical neurogenesis during early brain development. Sci Rep 2014; 4: 6010.
Gao Q, Liu L, Chen Y, Li H, Yang L, Wang Y et al. Synaptosome-related (SNARE) genes and their interactions contribute to the susceptibility and working memory of attention-deficit/hyperactivity disorder in males. Prog Neuropsychopharmacol Biol Psychiatry 2015; 57: 132–139.
Fekadu J, Rami A. Beclin-1 deficiency alters autophagosome formation, lysosome biogenesis and enhances neuronal vulnerability of ht22 hippocampal cells. Mol Neurobiol 2015; 53: 5500–5509.
Elmore S. Apoptosis: a review of programmed cell death. Toxicol Pathol 2007; 35: 495–516.
Haanen C, Vermes I. Apoptosis and inflammation. Mediators Inflamm 1995; 4: 5–15.
Awasaki T, Tatsumi R, Takahashi K, Arai K, Nakanishi Y, Ueda R et al. Essential role of the apoptotic cell engulfment genes draper and ced-6 in programmed axon pruning during Drosophila metamorphosis. Neuron 2006; 50: 855–867.
Snigdha S, Smith ED, Prieto GA, Cotman CW. Caspase-3 activation as a bifurcation point between plasticity and cell death. Neurosci Bull 2012; 28: 14–24.
Gilman CP, Mattson MP. Do apoptotic mechanisms regulate synaptic plasticity and growth-cone motility? Neuromolecular Med 2002; 2: 197–214.
Lo SC, Wang Y, Weber M, Larson JL, Scearce-Levie K, Sheng M. Caspase-3 deficiency results in disrupted synaptic homeostasis and impaired attention control. J Neurosci 2015; 35: 2118–2132.
Valbonesi S, Magri C, Traversa M, Faraone SV, Cattaneo A, Milanesi E et al. Copy number variants in attention-deficit hyperactive disorder: identification of the 15q13 deletion and its functional role. Psychiatr Genet 2015; 25: 59–70.
Xu P, Gu R, Broster LS, Wu R, Van Dam NT, Jiang Y et al. Neural basis of emotional decision making in trait anxiety. J Neurosci 2013; 33: 18641–18653.
Dalby KN, Tekedereli I, Lopez-Berestein G, Ozpolat B. Targeting the prodeath and prosurvival functions of autophagy as novel therapeutic strategies in cancer. Autophagy 2010; 6: 322–329.
Marino G, Niso-Santano M, Baehrecke EH, Kroemer G. Self-consumption: the interplay of autophagy and apoptosis. Nat Rev Mol Cell Biol 2014; 15: 81–94.
Rubinstein AD, Kimchi A. Life in the balance—a mechanistic view of the crosstalk between autophagy and apoptosis. J Cell Sci 2012; 125(Pt 22): 5259–5268.
Hou W, Han J, Lu C, Goldstein LA, Rabinowich H. Autophagic degradation of active caspase-8: a crosstalk mechanism between autophagy and apoptosis. Autophagy 2010; 6: 891–900.
Tanaka K, Whelan KA, Chandramouleeswaran PM, Kagawa S, Rustgi SL, Noguchi C et al. ALDH2 modulates autophagy flux to regulate acetaldehyde-mediated toxicity thresholds. Am J Cancer Res 2016; 6: 781–796.
Rodney GG, Pal R, Abo-Zahrah R. Redox regulation of autophagy in skeletal muscle. Free Radic Biol Med 2016; 98: 103–112.
Zhang L, Wang K, Lei Y, Li Q, Nice EC, Huang C. Redox signaling: potential arbitrator of autophagy and apoptosis in therapeutic response. Free Radic Biol Med 2015; 89: 452–465.
Huang J, Lam GY, Brumell JH. Autophagy signaling through reactive oxygen species. Antioxid Redox Signal 2011; 14: 2215–2231.
Cabal-Hierro L, Lazo PS. Signal transduction by tumor necrosis factor receptors. Cell Signal 2012; 24: 1297–1305.
Sriram K, O'Callaghan JP. Divergent roles for tumor necrosis factor-alpha in the brain. J Neuroimmune Pharmacol 2007; 2: 140–153.
International Multiple Sclerosis Genetics C, Wellcome Trust Case Control C, Sawcer S, Hellenthal G, Pirinen M, Spencer CC, Patsopoulos NA et al. Genetic risk and a primary role for cell-mediated immune mechanisms in multiple sclerosis. Nature 2011; 476: 214–219.
Instanes JT, Halmoy A, Engeland A, Haavik J, Furu K, Klungsoyr K. Attention-deficit/hyperactivity disorder in offspring of mothers with inflammatory and immune system diseases. Biol Psychiatry 2017; 81: 452–459.
Onnink AM, Zwiers MP, Hoogman M, Mostert JC, Dammers J, Kan CC et al. Deviant white matter structure in adults with attention-deficit/hyperactivity disorder points to aberrant myelination and affects neuropsychological performance. Prog Neuropsychopharmacol Biol Psychiatry 2015; 63: 14–22.
Kondo N, Takahashi A, Ono K, Ohnishi T. DNA damage induced by alkylating agents and repair pathways. J Nucleic Acids 2010; 2010: 543531.
Acknowledgments
Dr Faraone is supported by the K.G. Jebsen Centre for Research on Neuropsychiatric Disorders, University of Bergen, Bergen, Norway, the European Union’s Seventh Framework Programme for research, technological development and demonstration under grant agreement number 602805, the European Union’s Horizon 2020 research and innovation programme under grant agreement number 667302 and NIMH grants 5R01MH101519 and U01 MH109536-01. Dr Akutagava-Martins’ contribution was supported by the Conselho Nacional de Desenvolvimento CientÃfico e Tecnológico (CNPq), Brazil.
Author information
Authors and Affiliations
Corresponding author
Ethics declarations
Conflict of Interest
In the past year, Dr Faraone received income, potential income, travel expenses continuing education support and/or research support from Lundbeck, Rhodes, Arbor, KenPharm, Ironshore, Shire, Akili Interactive Labs, CogCubed, Alcobra, VAYA, Sunovion, Genomind and Neurolifesciences. With his institution, he has US patent US20130217707 A1 for the use of sodium-hydrogen exchange inhibitors in the treatment of ADHD. In previous years, he received support from: Shire, Neurovance, Alcobra, Otsuka, McNeil, Janssen, Novartis, Pfizer and Eli Lilly. Dr Faraone receives royalties from books published by Guilford Press: Straight Talk about Your Child’s Mental Health, Oxford University Press: Schizophrenia: The Facts and Elsevier: ADHD: Non-Pharmacologic Interventions. He is principal investigator of www.adhdinadults.com. All other authors declare no conflict of interest.
Electronic supplementary material
Rights and permissions
About this article
Cite this article
Hess, J.L., Akutagava-Martins, G.C., Patak, J.D. et al. Why is there selective subcortical vulnerability in ADHD? Clues from postmortem brain gene expression data. Mol Psychiatry 23, 1787–1793 (2018). https://doi.org/10.1038/mp.2017.242
Received:
Revised:
Accepted:
Published:
Issue Date:
DOI: https://doi.org/10.1038/mp.2017.242
This article is cited by
-
Transcriptomic risk scores for attention deficit/hyperactivity disorder
Molecular Psychiatry (2023)
-
Autophagy, apoptosis, and neurodevelopmental genes might underlie selective brain region vulnerability in attention-deficit/hyperactivity disorder
Molecular Psychiatry (2021)
-
Three genetic–environmental networks for human personality
Molecular Psychiatry (2021)
-
Structural brain imaging studies offer clues about the effects of the shared genetic etiology among neuropsychiatric disorders
Molecular Psychiatry (2021)
-
ENIGMA and global neuroscience: A decade of large-scale studies of the brain in health and disease across more than 40 countries
Translational Psychiatry (2020)